
I am a Information Treasure Hunter. I was sent to earth to teach Love and Kindness
A Mass Murderer.
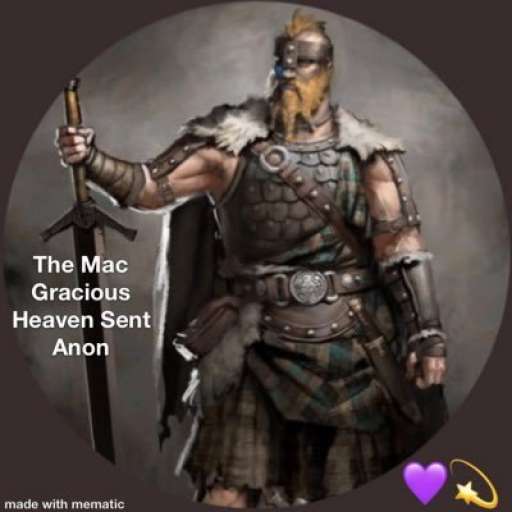
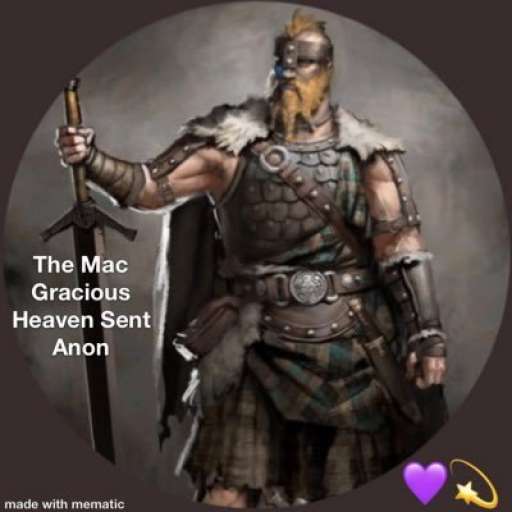
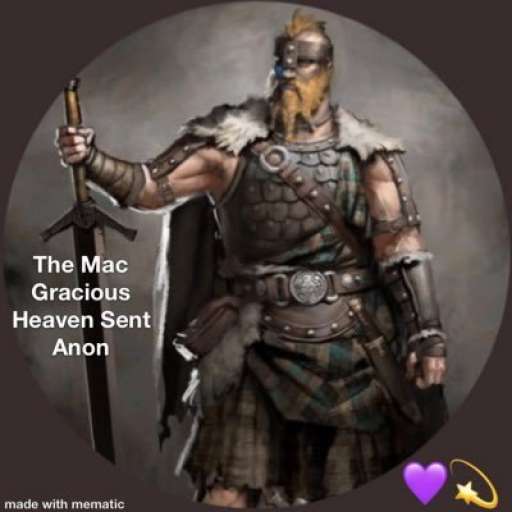
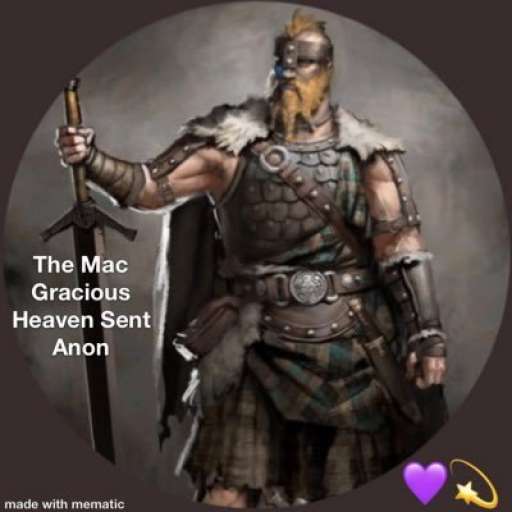
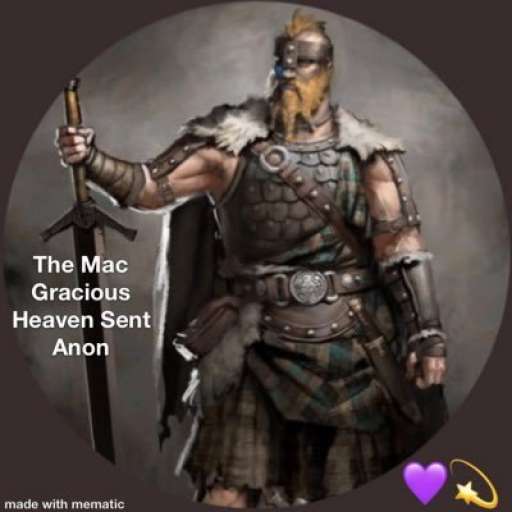
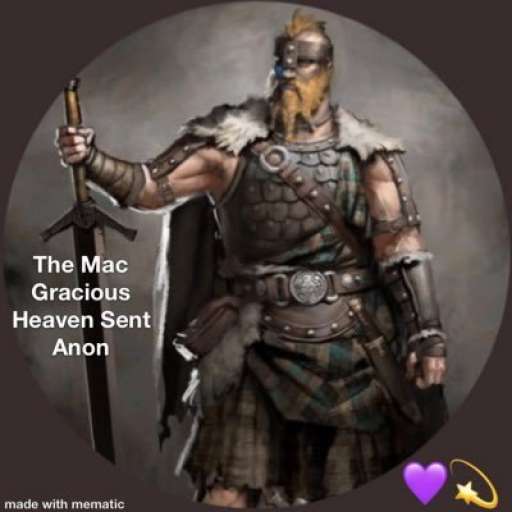
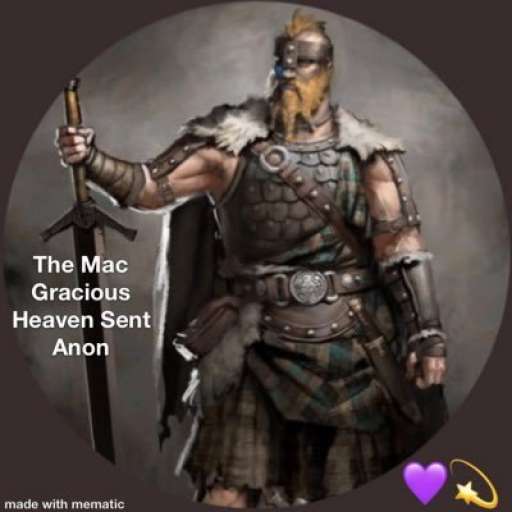
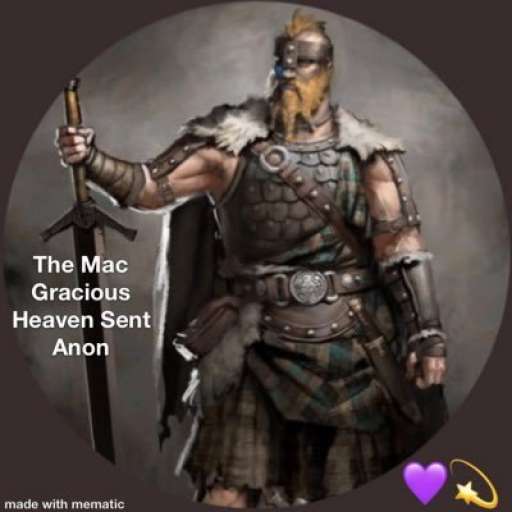
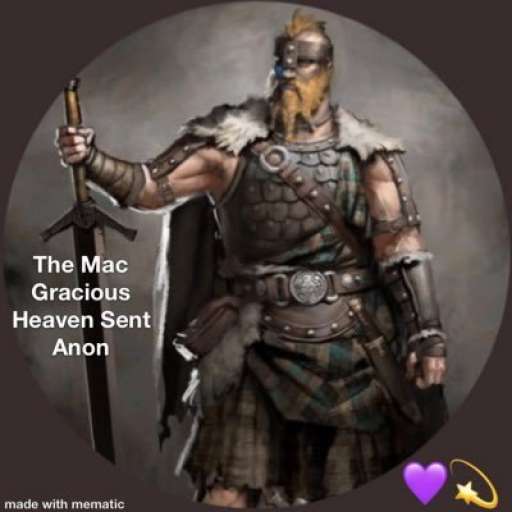
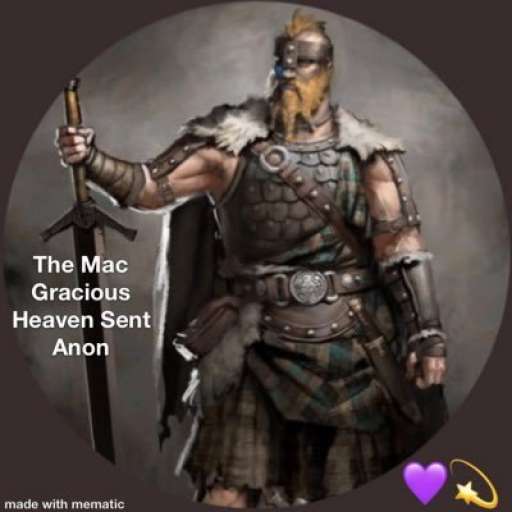
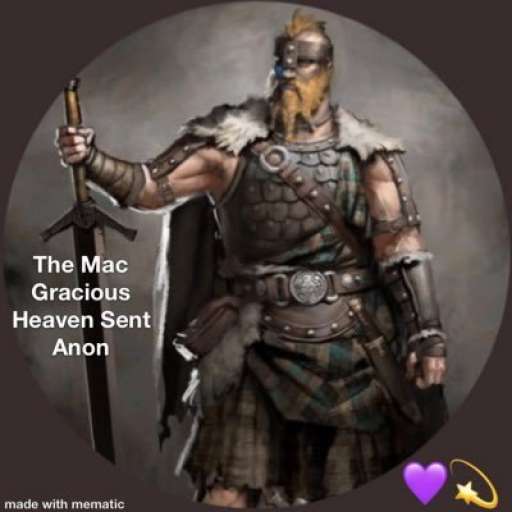
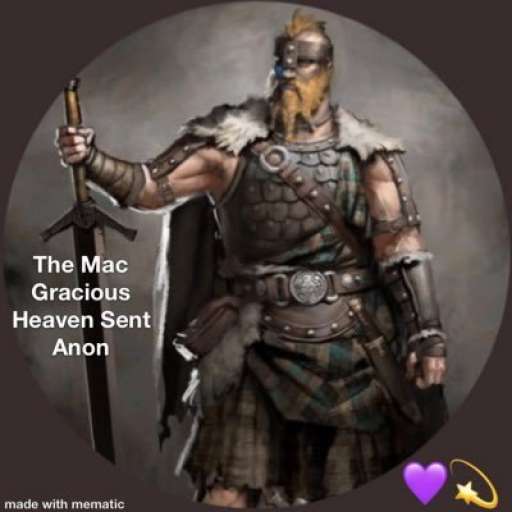
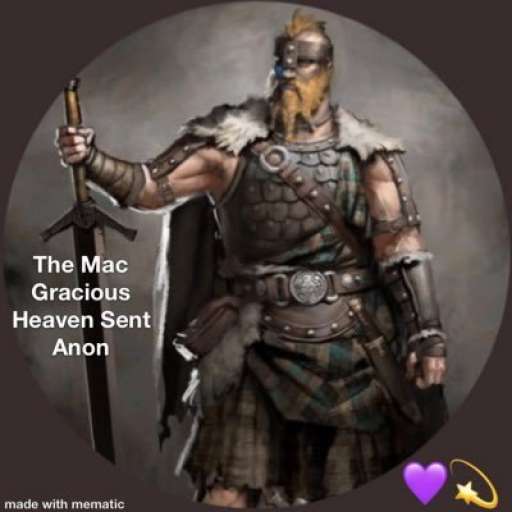
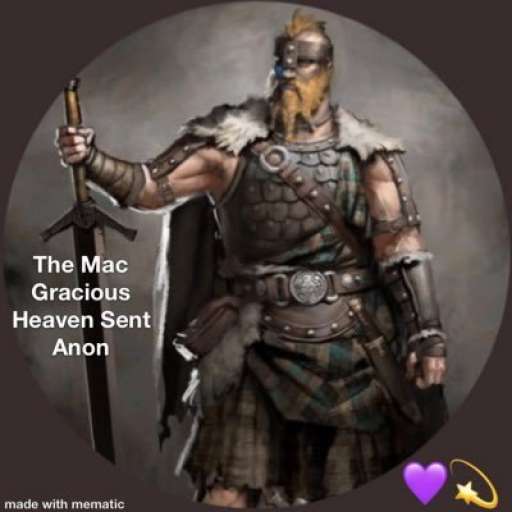
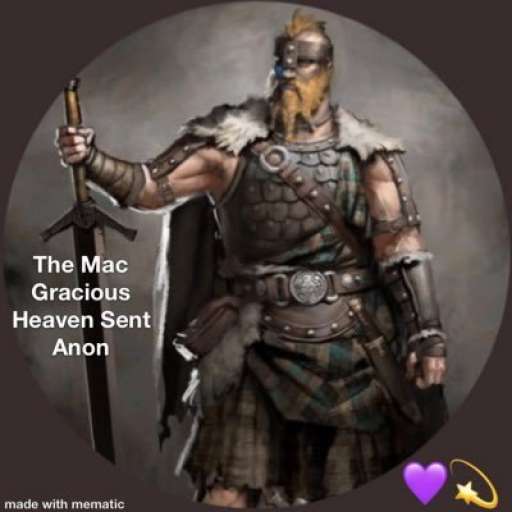
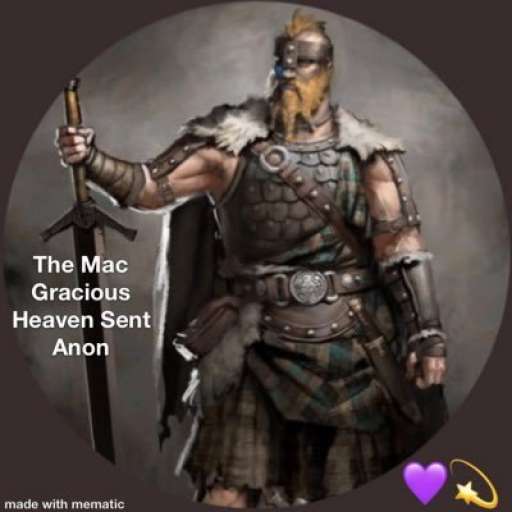
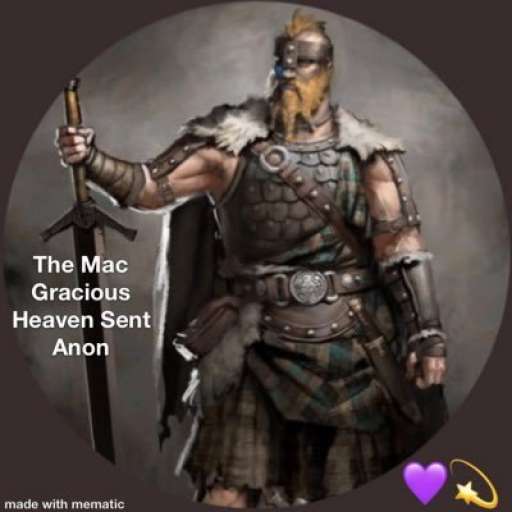
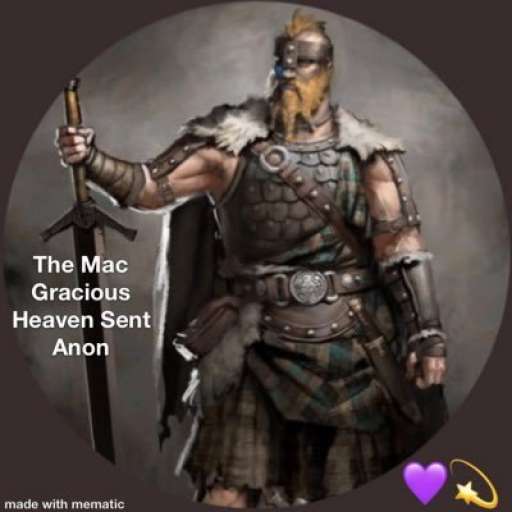
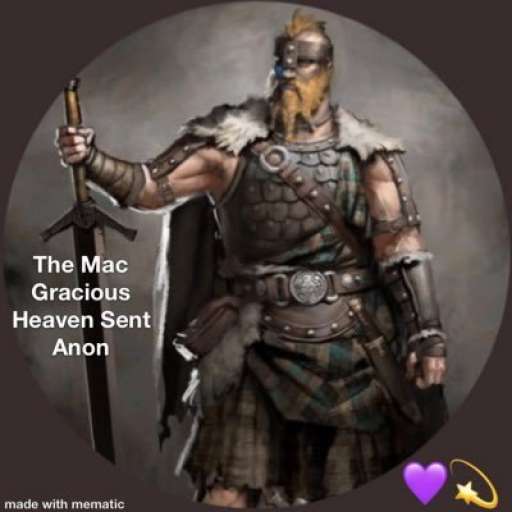
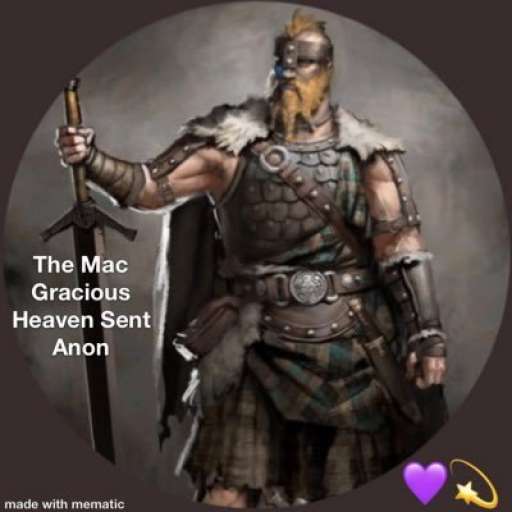
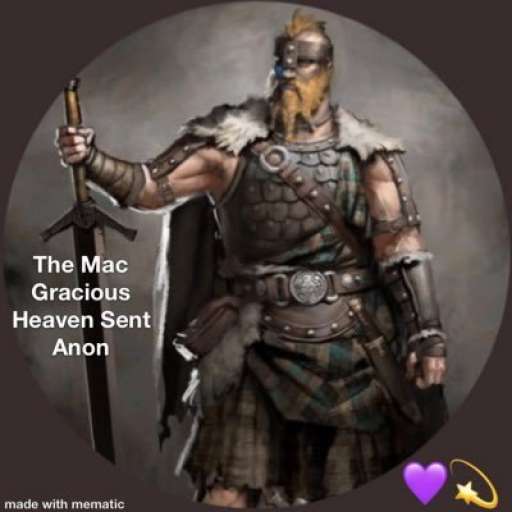
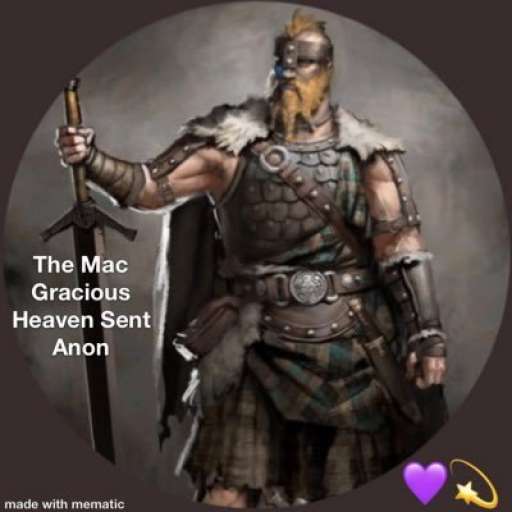
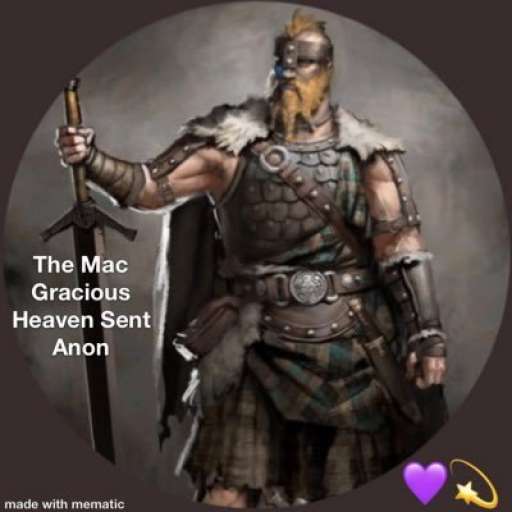
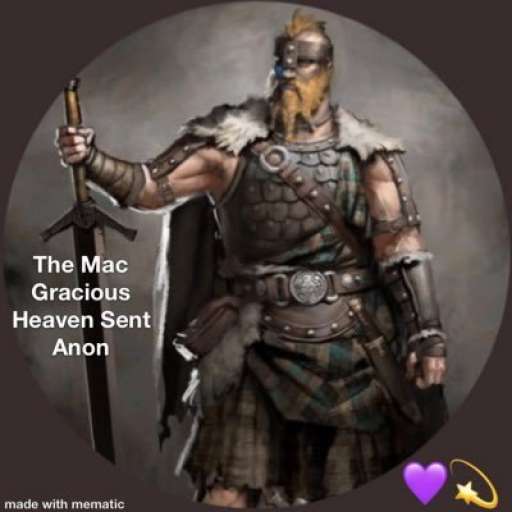
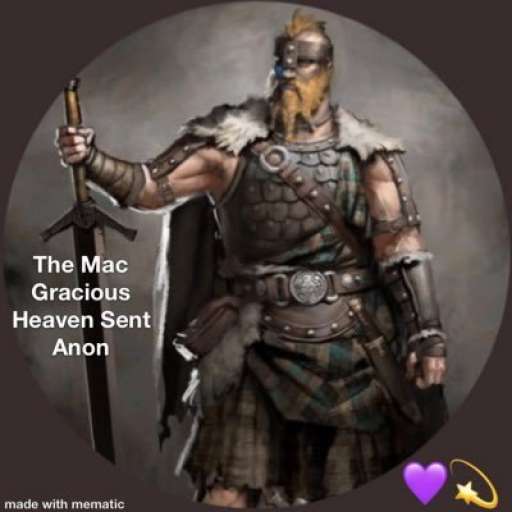
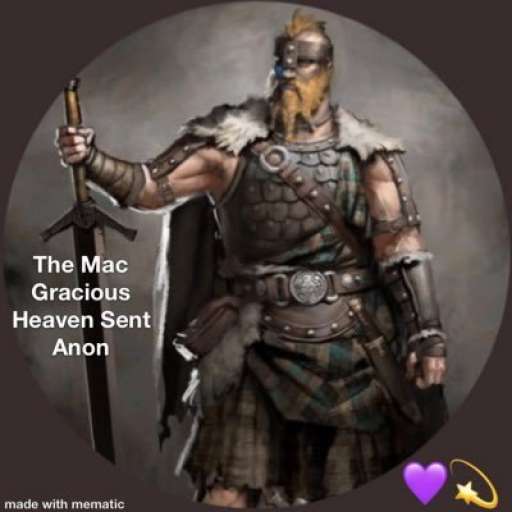
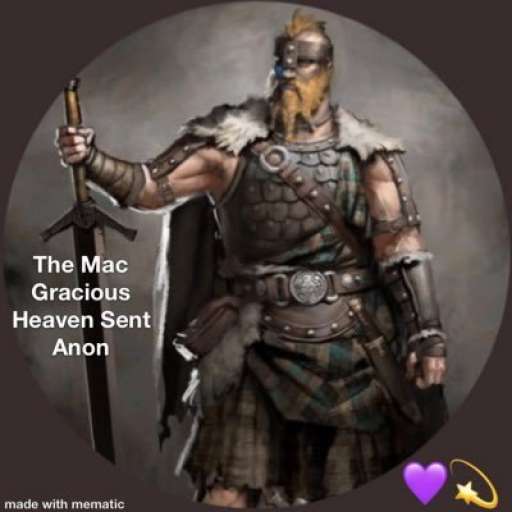
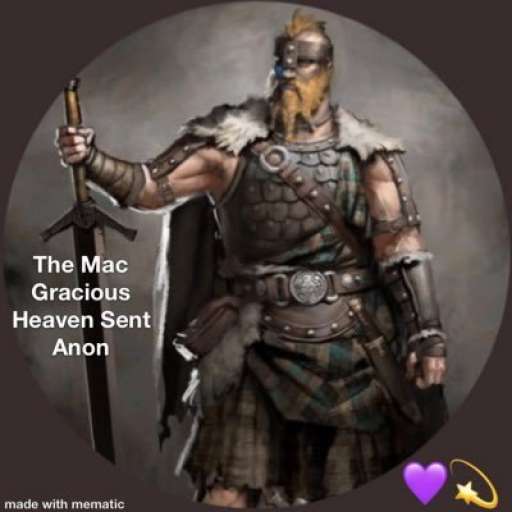
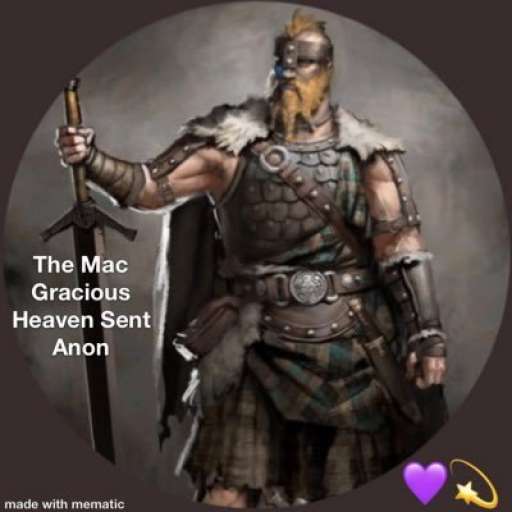
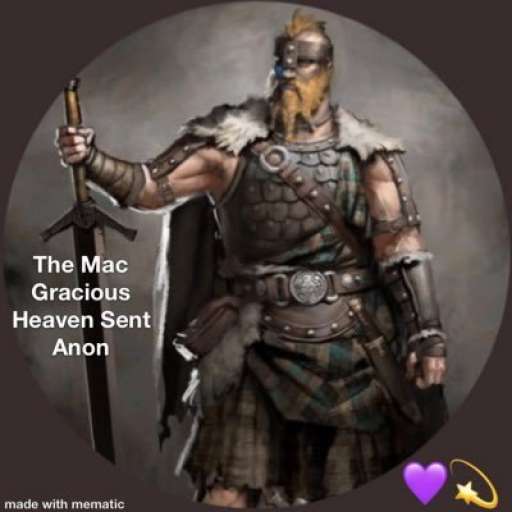
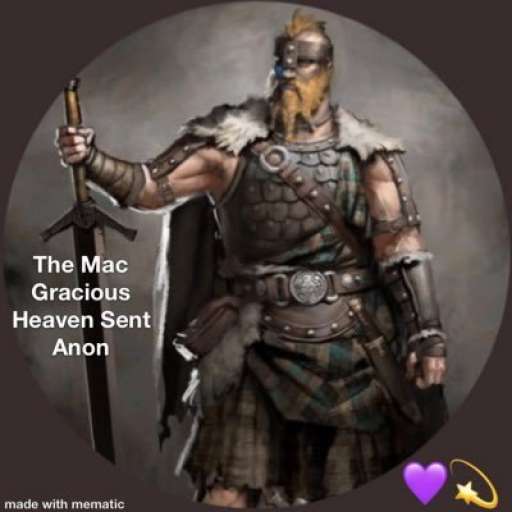
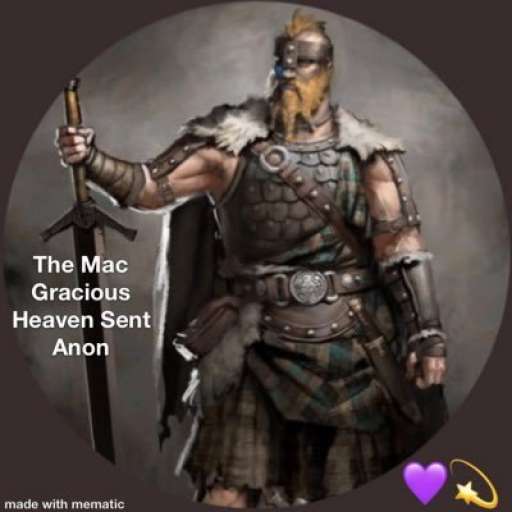
Lipid peroxidation markers are elevated in autism, indicating that oxidative stress is increased in this disease. Levels of major antioxidant serum proteins, namely transferrin (iron-binding protein) and ceruloplasmin (copper-binding protein), are decreased in children with autism. There is a positive correlation between reduced levels of these proteins and loss of previously acquired language skills in children with autism. The alterations in ceruloplasmin and transferrin levels may lead to abnormal iron and copper metabolism in autism. The membrane phospholipids, the prime target of ROS, are also altered in autism. The levels of phosphatidylethanolamine (PE) are decreased, and phosphatidylserine (PS) levels are increased in the erythrocyte membrane of children with autism as compared to their unaffected siblings. Several studies have suggested alterations in the activities of antioxidant enzymes such as superoxide dismutase, glutathione peroxidase, and catalase in autism.
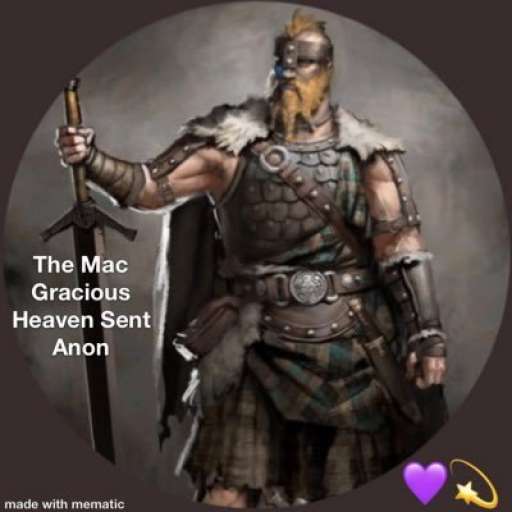
Additionally, altered glutathione levels and homocysteine/methionine metabolism, increased inflammation, excitotoxicity, as well as mitochondrial and immune dysfunction have been suggested in autism. Furthermore, environmental and genetic factors may increase vulnerability to oxidative stress in autism. Taken together, these studies suggest increased oxidative stress in autism that may contribute to the development of this disease. A mechanism linking oxidative stress with membrane lipid abnormalities, inflammation, aberrant immune response, impaired energy metabolism and excitotoxicity, leading to clinical symptoms and pathogenesis of autism is proposed.
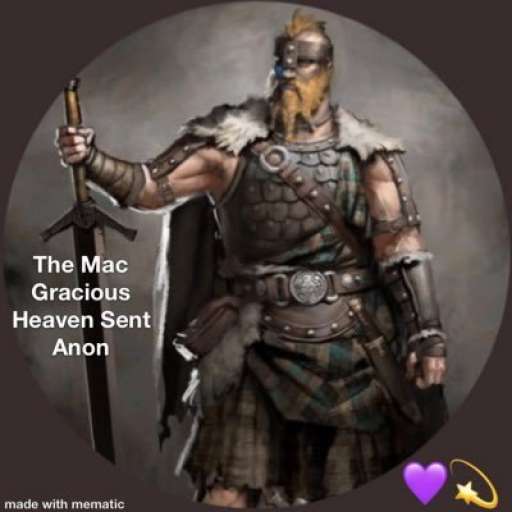
2. ROS-related redox mechanisms of nano-iron metal and nano-iron oxides
Successive one-electron or two-electron reduction of molecular oxygen to water in the aqueous solution yields a series of ROS such as superoxide radicals (O2−/HO2radical dot), hydrogen peroxide (H2O2), and hydroxyl radicals (OHradical dot). Nano-iron metal and nano-iron oxides can be involved in these redox reactions as the reactant or catalyst via a homogeneous or heterogeneous means, based on dissolved iron species or solid surfaces, respectively. The standard reduction potentials (E0) of redox pairs covered in this review are cited from previous studies [13], [14], [15].
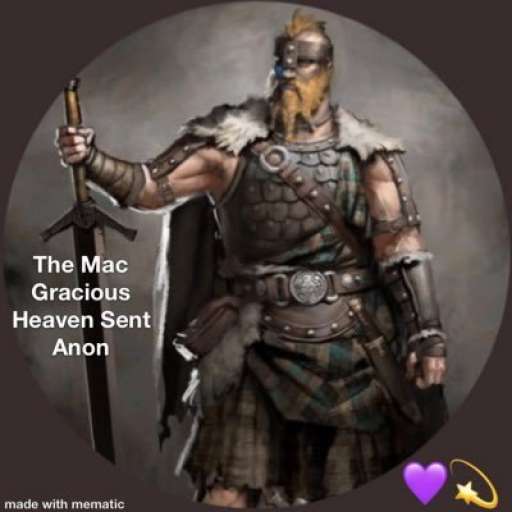
2.1. Homogeneous reactions
(1) The classic homogeneous Fenton reaction, which involves one-electron reduction of hydrogen peroxide by soluble ferrous iron species, generates hydroxyl radicals (E0 = +2.3 V) that are powerful enough to oxidize most organic molecules:
(1)
By convention, Fe(II) and Fe(III) represent all ferrous and ferric iron species, respectively.
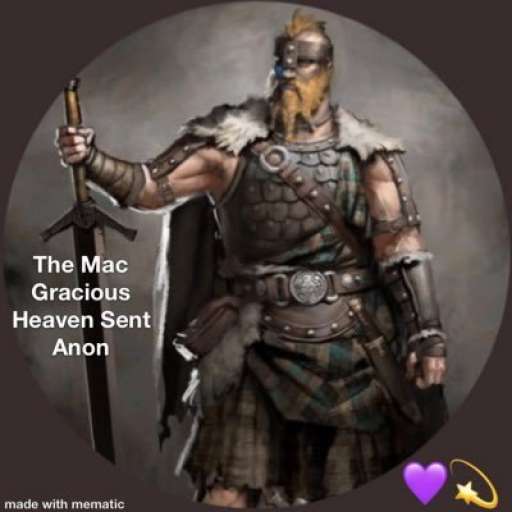
(2) The non-radical mechanism for the homogeneous Fenton reaction involves the generation of ferryl-oxo complexes (E0 = +0.9 V), less powerful oxidants compared to hydroxyl radicals, via two-electron reduction of hydrogen peroxide with soluble ferrous iron species:
(2)
Based on the distinct oxidizing power of hydroxyl radicals and ferryl-oxo complexes, many studies have found that radical and non-radical Fenton reactions are competing reactions with a pH-dependent partitioning [16], [17], [18].
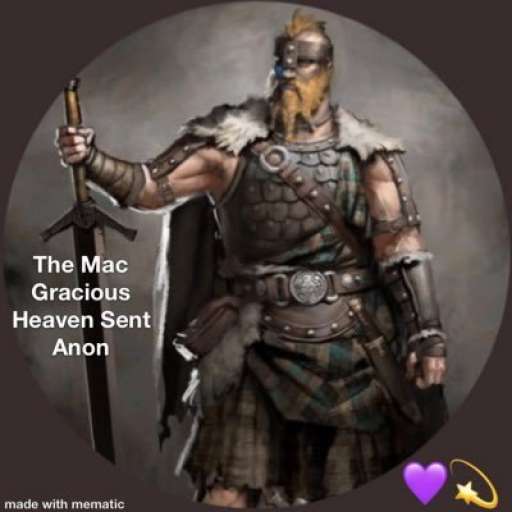
(3) The homogeneous Fenton-like reactions, which involve the generation of superoxide radicals, hydroxyl radicals, or ferryl-oxo complexes from hydrogen peroxide and soluble ferric iron species, consist of two steps; a slow one-electron reduction of ferric iron by hydrogen peroxide and a rapid generation of powerful oxidants via reaction (1), (2):
(3)
(1)
(2)
(3) The Haber-Weiss reaction, which involves generation of hydroxyl radicals from hydrogen peroxide and superoxide, can be catalyzed by soluble ferric iron species through two steps:
(4)
(1)
(4) The homogeneous Fe(II) autoxidation in the presence of molecular oxygen in the aqueous solution via single-step two-electron transfer or stepwise one-electron transfer reactions can generate oxidants of ferryl-oxo complexes or a series of ROS [19], [20]:
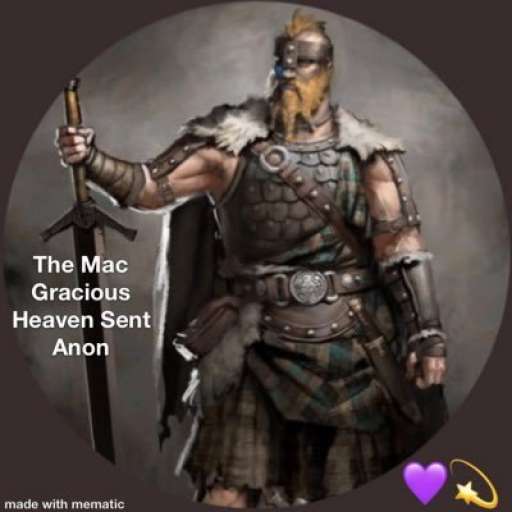
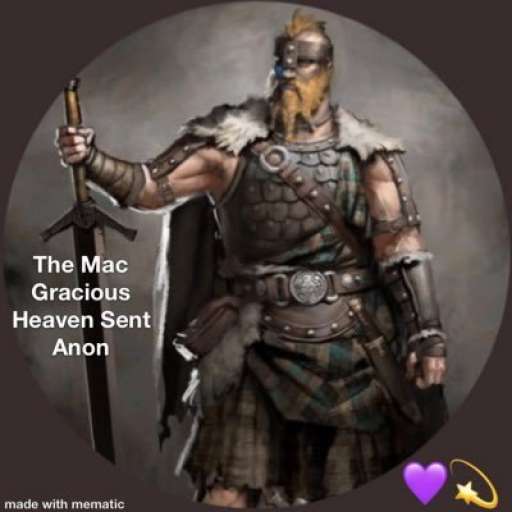
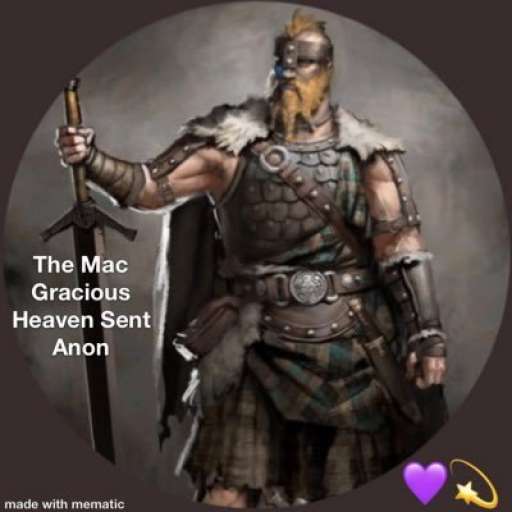
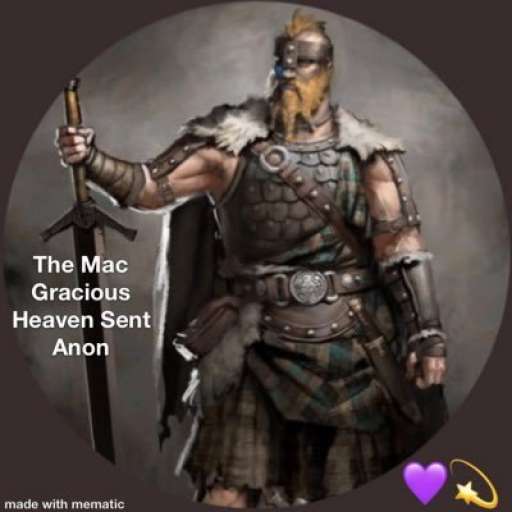
It has long been recognized that iron oxides can initiate heterogeneous redox reactions at the aqueous-solid interface. Zalma et al [22] reported that iron oxides including akaganeite, magnetite, crocidolite, and hematite were active in producing hydroxyl radicals in the presence of hydrogen peroxide by redox reactions initiated at the solid surface. Lin and Gurol [23] found that the kinetic model of catalytic decomposition of hydrogen peroxide by the granular goethite in aqueous solution at pH 7 was similar to the classical Langmuir-Hinshelwood rate model, which usually describes heterogeneous surface reactions, and proposed a redox mechanism of iron oxides based on the surface-complexation chemistry of surface-bound ferric [≡Fe(III)] and ferrous [≡Fe(II)] iron: