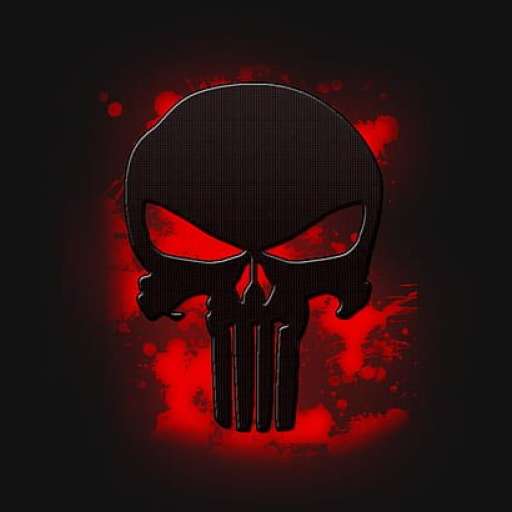
4734
17-Sep-2020 4:40:53 PM EDT
8kun/qresearch10685190
Focus on content [information].
Research for yourself.
gatekeeper
noun
a person that controls access, as to information, often acting as an arbiter of quality or legitimacy:
an open internet allows innovators to bypass traditional gatekeepers and promote their work on its own merit.
a guardian; monitor
Deploy camouflage.
Drop all references re: 'Q' 'Qanon' etc. to avoid ban/termination _censorship install.
Algos [sniffers] bypass.
Keep charging, Midnight Riders!
[Revere's 'Midnight' Ride]
Delivery of free-information.
[bypa
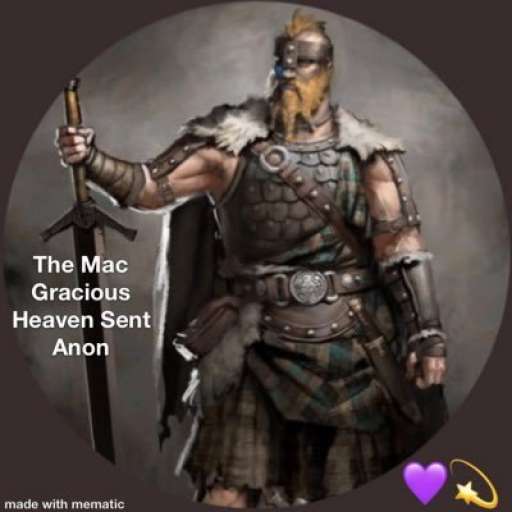
The process of making
or declaring a person legitimate.
Le gi ta ma cy
opposite: Joe Biden is delegitamate.
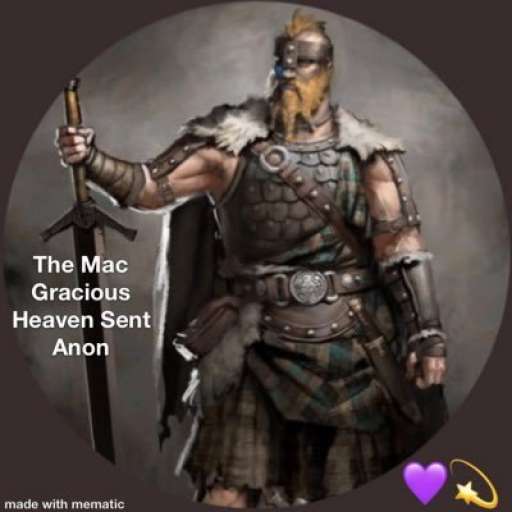
algos (sniffers) bypass?
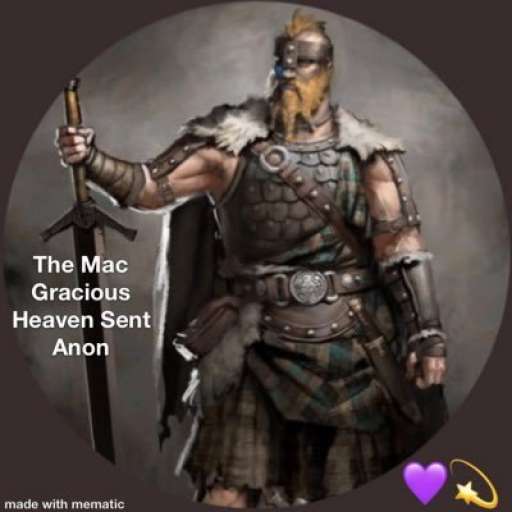
a road that passes around something, such as a residential area· a circumvention· a section of pipe that conducts a fluid around some other fixture an electrical shunt (medicine) an alternative passage created to divert a bodily fluid around a damaged organ; the surgical procedure to construct such a bypass·to avoid an obstacle etc, by constructing ...
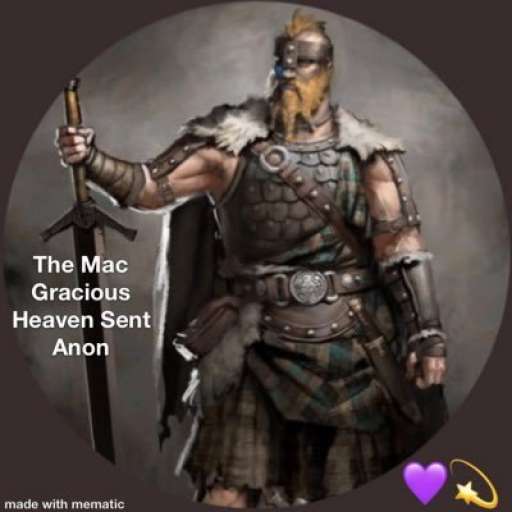
circumvention (countable and uncountable, plural circumventions)
The act of evading by going around (bypassing).
The act of prevailing over another by fraud or deception
Synonyms: deception, fraud, imposture, delusion
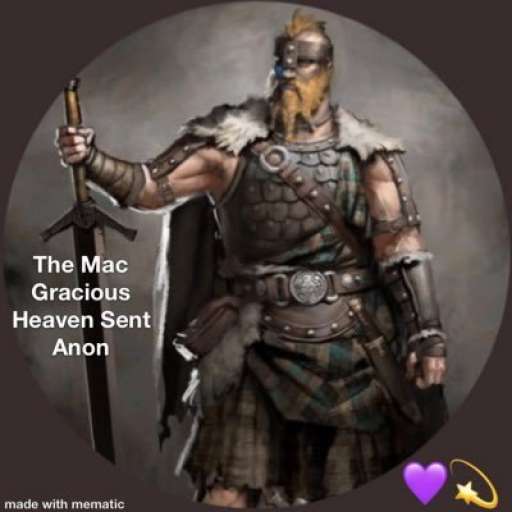
circumvent (third-person singular simple present circumvents, present participle circumventing, simple past and past participle circumvented)
(transitive) to avoid or get around something; to bypass
(transitive) to surround or besiege
(transitive) to outwit or outsmart
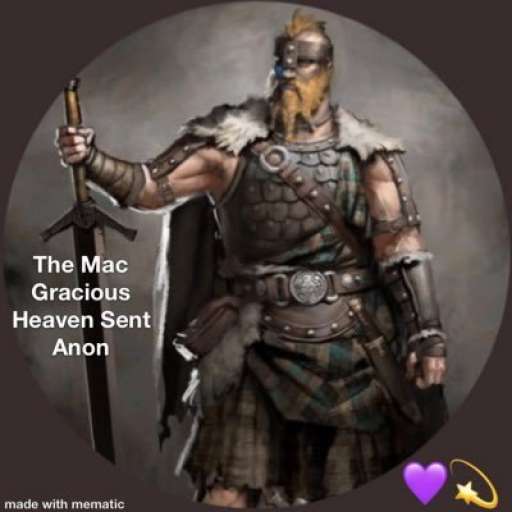
circumventable (comparative more circumventable, superlative most circumventable)
Capable of being circumvented.
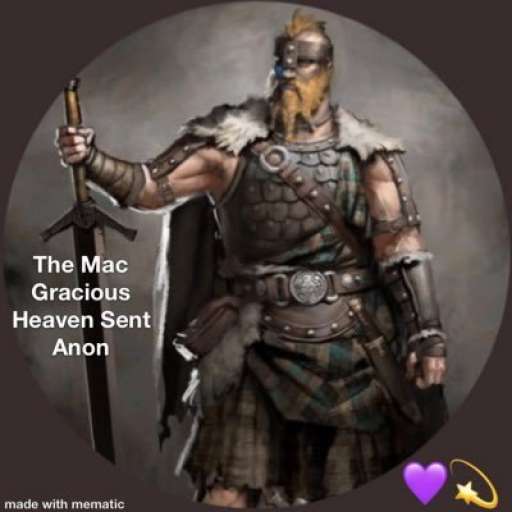
outwit (third-person singular simple present outwits, present participle outwitting, simple past and past participle outwitted)
(transitive) To get the better of; to outsmart, to beat in a competition of wits.
Synonyms
outfox, outguess, outsmart, overreach
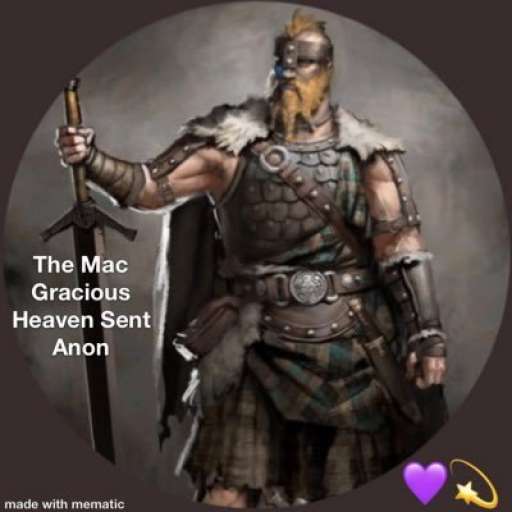
outsmart (third-person singular simple present outsmarts, present participle outsmarting, simple past and past participle outsmarted)
(transitive) To beat in a competition of wits.
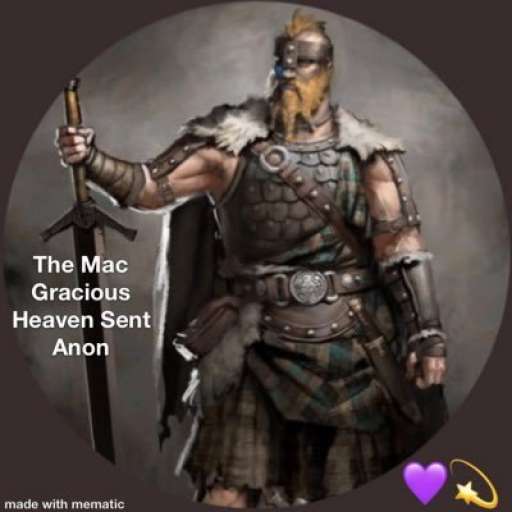
The design of smart dust sensors is a new frontier in the miniaturisation of lab-on-a-chip devices, since the traditional model of a passive cartridge inserted into a hand-held or desktop device has to be abandoned. The smart dust concept originated in the 1990s, but the size of the assembled microelectronic devices exceeded a cubic millimetre.
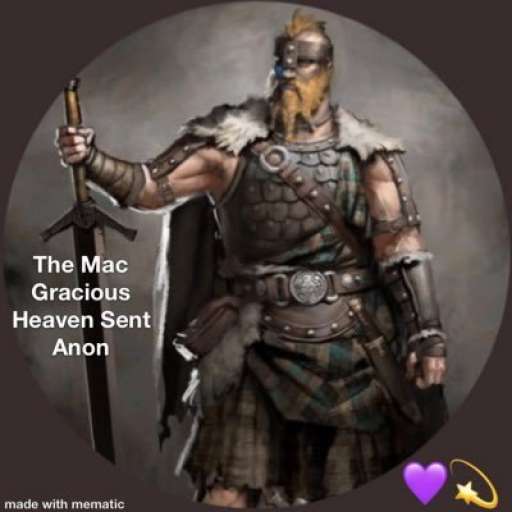
Biosensors can be miniaturized by either injecting smaller volumes into micro- and nanofluidic devices or immersing increasingly sophisticated particles known as 'smart dust' into the sample.
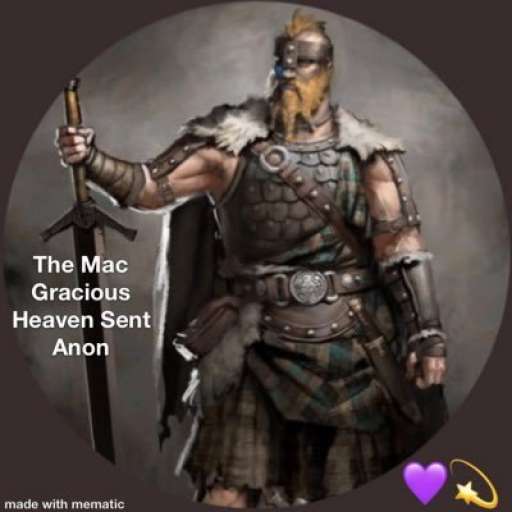
Smartdust is a system of many tiny microelectromechanical systems (MEMS) such as sensors, robots, or other devices, that can detect, for example, light, temperature, vibration, magnetism, or chemicals.
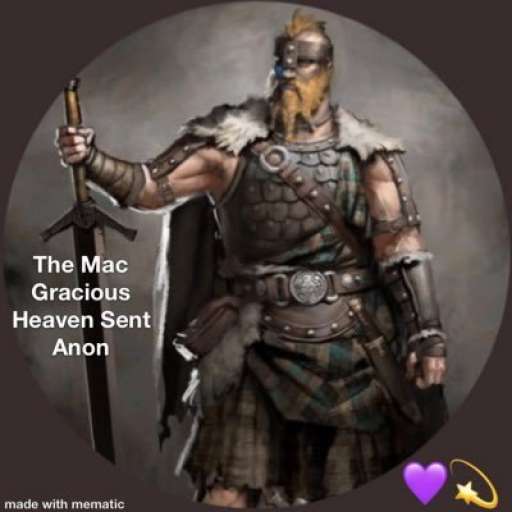
Bio-MEMS is an abbreviation for biomedical (or biological) microelectromechanical systems. Bio-MEMS have considerable overlap, and is sometimes considered synonymous, with lab-on-a-chip (LOC) and micro total analysis systems (μTAS). Bio-MEMS is typically more focused on mechanical parts and microfabrication technologies made suitable for biological applications. On the other hand, lab-on-a-chip is concerned with miniaturization and integration of laboratory processes and experiments into single (often microfluidic) chips.
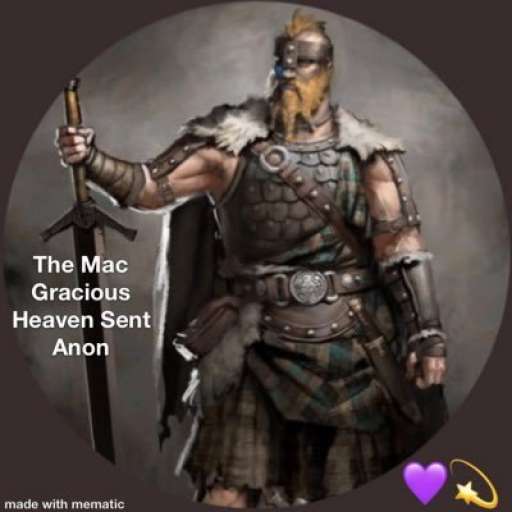
In this definition, lab-on-a-chip devices do not strictly have biological applications, although most do or are amenable to be adapted for biological purposes. Similarly, micro total analysis systems may not have biological applications in mind, and are usually dedicated to chemical analysis. A broad definition for bio-MEMS can be used to refer to the science and technology of operating at the microscale for biological and biomedical applications, which may or may not include any electronic or mechanical functions.
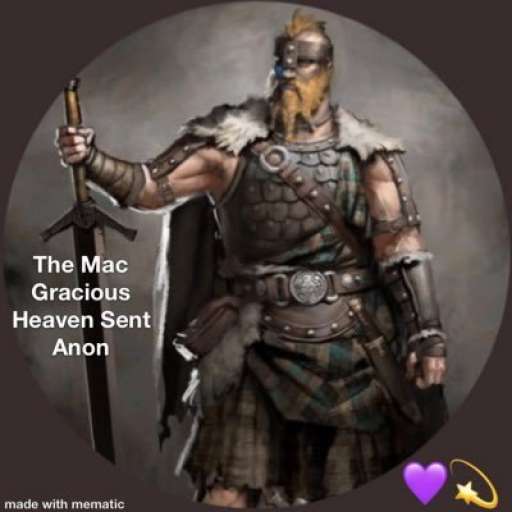
The interdisciplinary nature of bio-MEMS combines material sciences, clinical sciences, medicine, surgery, electrical engineering, mechanical engineering, optical engineering, chemical engineering, and biomedical engineering. Some of its major applications include genomics, proteomics, molecular diagnostics, point-of-care diagnostics, tissue engineering, single cell analysis and implantable microdevices.
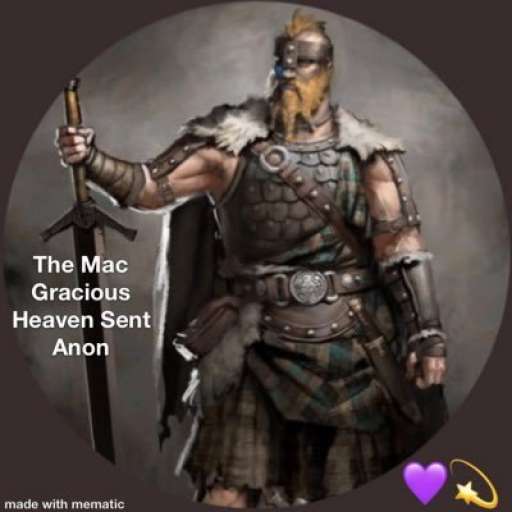
Optical engineering is the field of study that focuses on applications of optics. Optical engineering deals with design of optical instruments such as lenses, microscopes, telescopes, and other equipment that utilizes the properties of light using physics and chemistry.
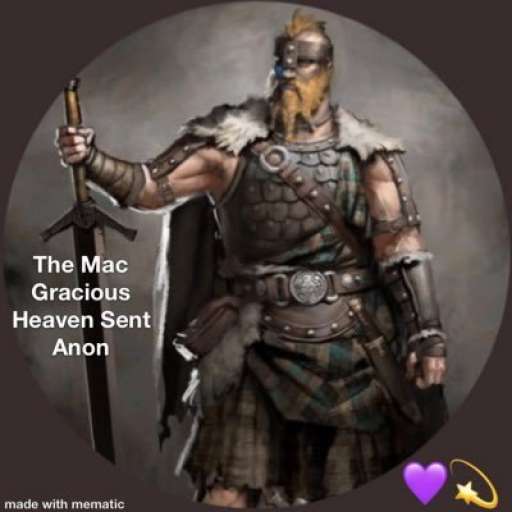
Optical physics is the study of the generation of electromagnetic radiation, the properties of that radiation, and the interaction of that radiation with matter, especially its manipulation and control. It differs from general optics and optical engineering in that it is focused on the discovery and application of new phenomena.
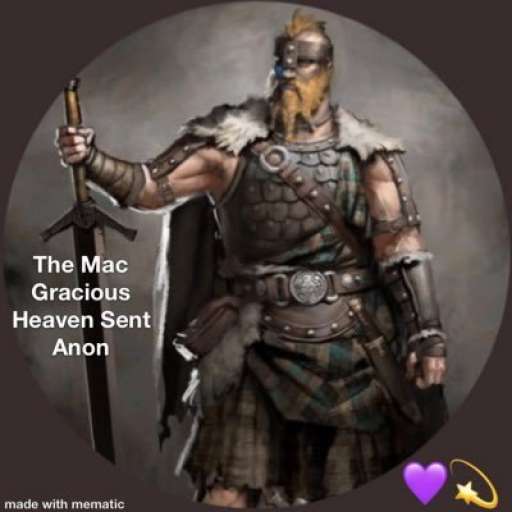
There is no strong distinction, however, between optical physics, applied optics, and optical engineering, since the devices of optical engineering and the applications of applied optics are necessary for basic research in optical physics, and that research leads to the development of new devices and applications. Often the same people are involved in both the basic research and the applied technology development, for example the experimental demonstration of electromagnetically induced transparency by S. E. Harris and of slow light by Harris and Lene Vestergaard Hau.
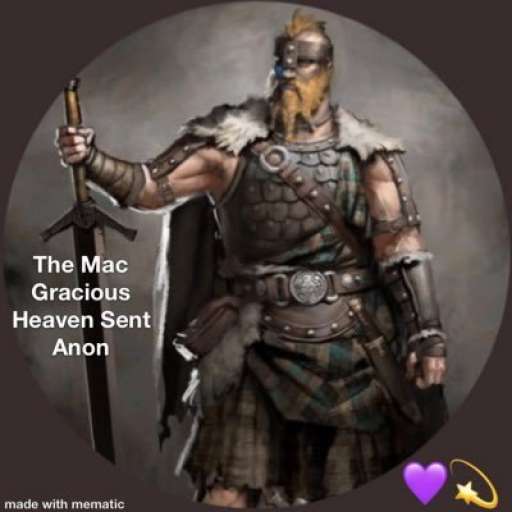
Researchers in optical physics use and develop light sources that span the electromagnetic spectrum from microwaves to X-rays. The field includes the generation and detection of light, linear and nonlinear optical processes, and spectroscopy. Lasers and laser spectroscopy have transformed optical science.
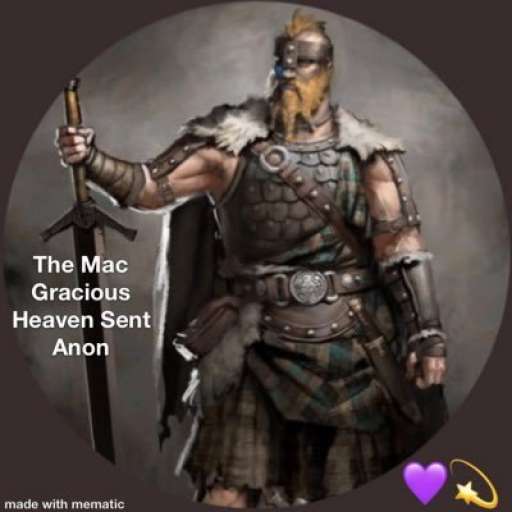
Major study in optical physics is also devoted to quantum optics and coherence, and to femtosecond optics. In optical physics, support is also provided in areas such as the nonlinear response of isolated atoms to intense, ultra-short electromagnetic fields, the atom-cavity interaction at high fields, and quantum properties of the electromagnetic field.
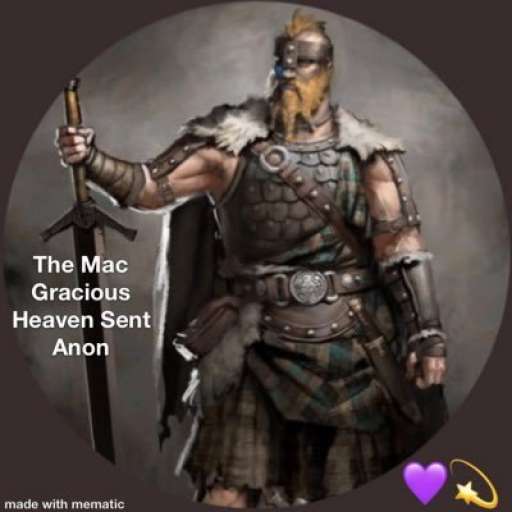
Other important areas of research include the development of novel optical techniques for nano-optical measurements, diffractive optics, low-coherence interferometry, optical coherence tomography, and near-field microscopy. Research in optical physics places an emphasis on ultrafast optical science and technology. The applications of optical physics create advancements in communications, medicine, manufacturing, and even entertainment.
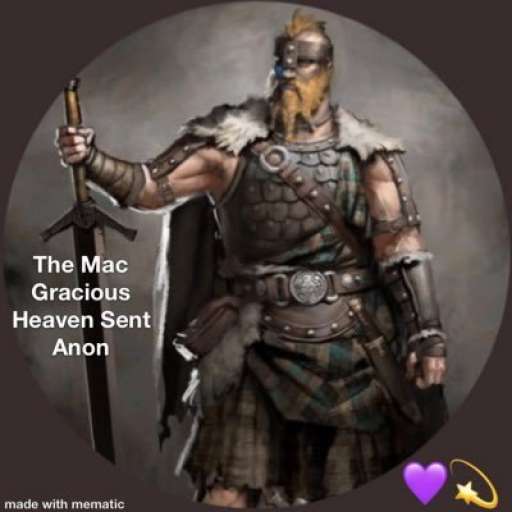
Electrons form notional shells around the nucleus. These are naturally in a ground state but can be excited by the absorption of energy from light (photons), magnetic fields, or interaction with a colliding particle (typically other electrons).
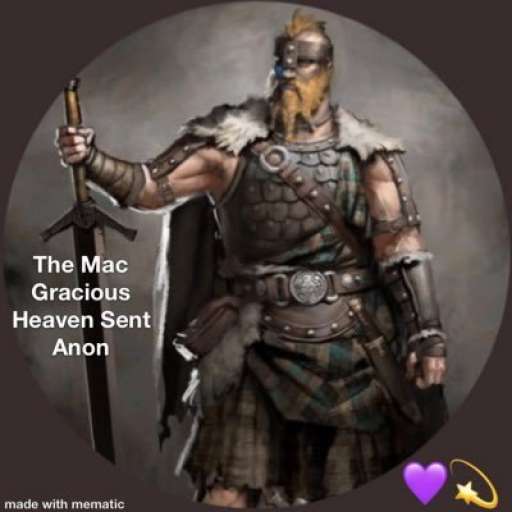
Electrons that populate a shell are said to be in a bound state. The energy necessary to remove an electron from its shell (taking it to infinity) is called the binding energy. Any quantity of energy absorbed by the electron in excess of this amount is converted to kinetic energy according to the conservation of energy. The atom is said to have undergone the process of ionization.
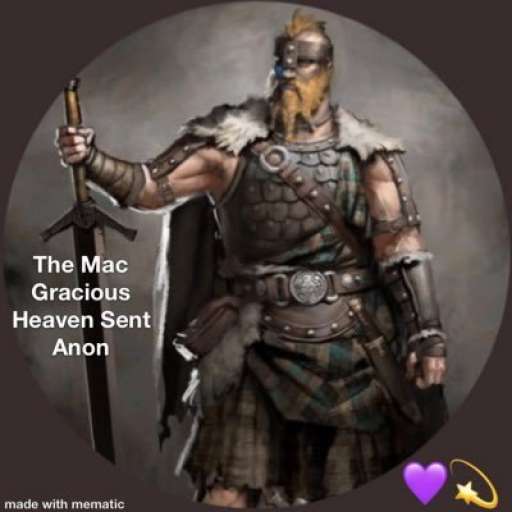
In the event that the electron absorbs a quantity of energy less than the binding energy, it may transition to an excited state or to a virtual state. After a statistically sufficient quantity of time, an electron in an excited state will undergo a transition to a lower state via spontaneous emission.
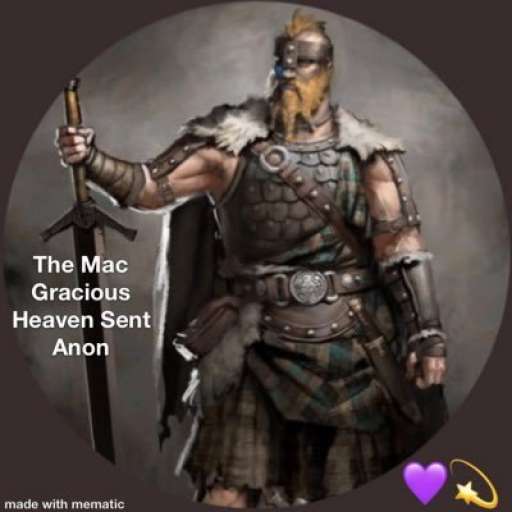
The change in energy between the two energy levels must be accounted for (conservation of energy). In a neutral atom, the system will emit a photon of the difference in energy. However, if the lower state is in an inner shell, a phenomenon known as the Auger effect may take place where the energy is transferred to another bound electrons causing it to go into the continuum. This allows one to multiply ionize an atom with a single photon.
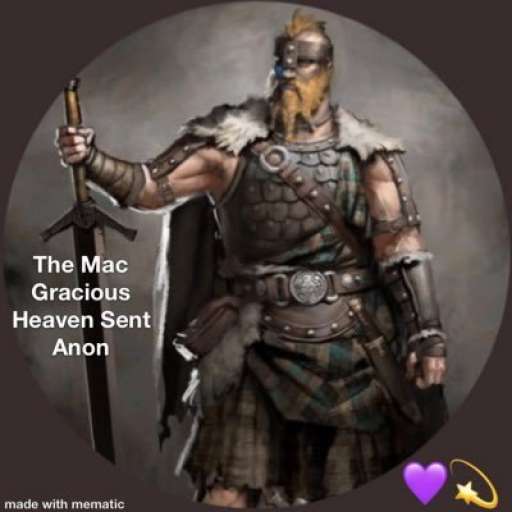
There are strict selection rules as to the electronic configurations that can be reached by excitation by light—however there are no such rules for excitation by collision processes.
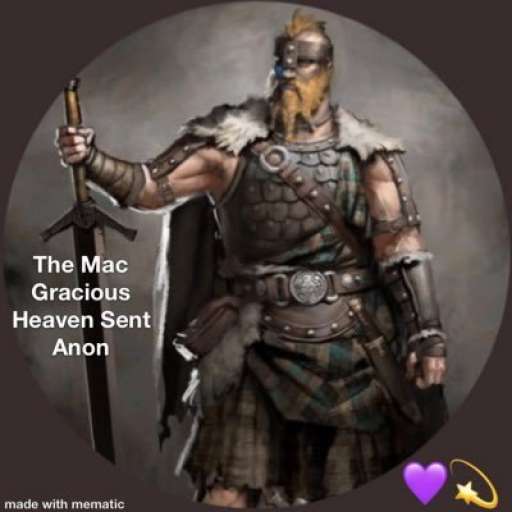
Photon polarization is the quantum mechanical description of the classical polarized sinusoidal plane electromagnetic wave. An individual photon can be described as having right or left circular polarization, or a superposition of the two. Equivalently, a photon can be described as having horizontal or vertical linear polarization, or a superposition of the two.
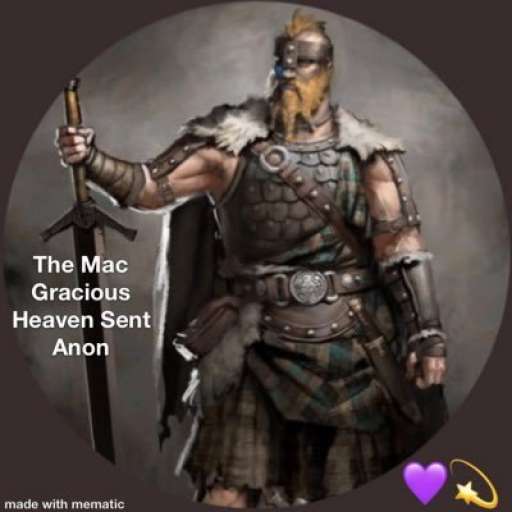
The connection with quantum mechanics is made through the identification of a minimum packet size, called a photon, for energy in the electromagnetic field. The identification is based on the theories of Planck and the interpretation of those theories by Einstein. The correspondence principle then allows the identification of momentum and angular momentum (called spin), as well as energy, with the photon.
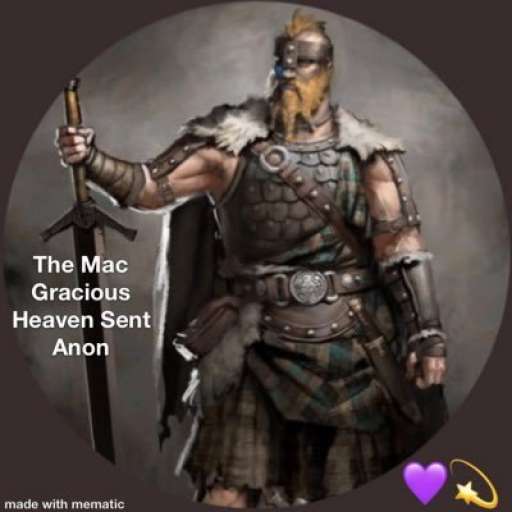
An ideal birefringent crystal transforms the polarization state of an electromagnetic wave without loss of wave energy. Birefringent crystals therefore provide an ideal test bed for examining the conservative transformation of polarization states. Even though this treatment is still purely classical, standard quantum tools such as unitary and Hermitian operators that evolve the state in time naturally emerge.
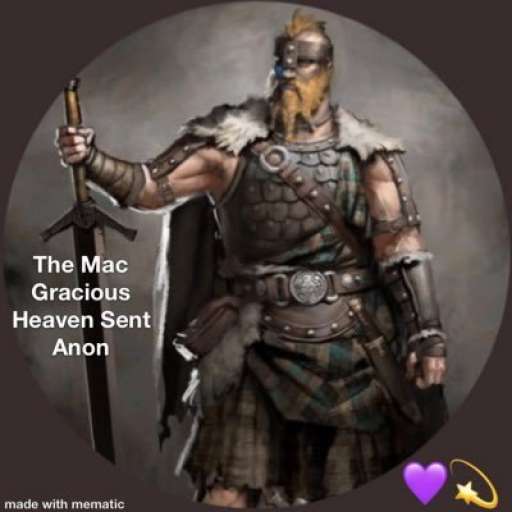
A birefringent crystal is a material that has an optic axis with the property that the light has a different index of refraction for light polarized parallel to the axis than it has for light polarized perpendicular to the axis. Light polarized parallel to the axis are called "extraordinary rays" or "extraordinary photons", while light polarized perpendicular to the axis are called "ordinary rays" or "ordinary photons".
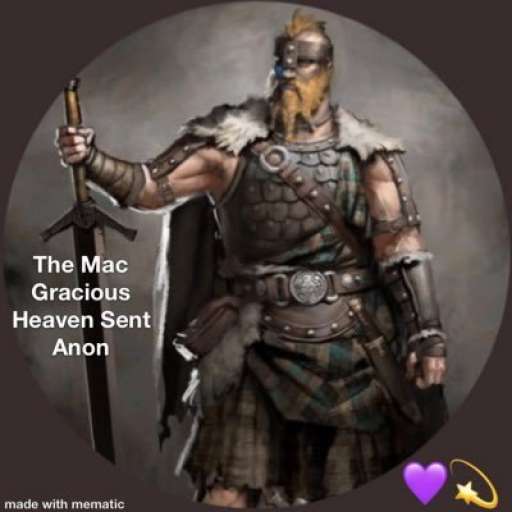
If a linearly polarized wave impinges on the crystal, the extraordinary component of the wave will emerge from the crystal with a different phase than the ordinary component. In mathematical language, if the incident wave is linearly polarized at an angle
θ with respect to the optic axis, the incident state vector can be written
Birefringence is the optical property of a material having a refractive index that depends on the polarization and propagation direction of light. These optically anisotropic materials are said to be birefringent (or birefractive). The birefringence is often quantified as the maximum difference between refractive indices exhibited by the material. Crystals with non-cubic crystal structures are often birefringent, as are plastics under mechanical stress.
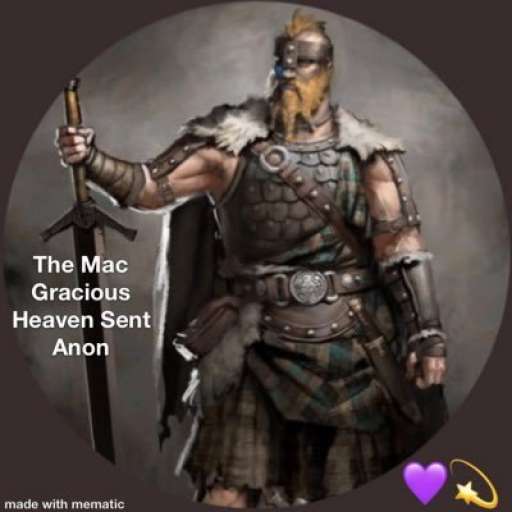
Birefringence is responsible for the phenomenon of double refraction whereby a ray of light, when incident upon a birefringent material, is split by polarization into two rays taking slightly different paths.
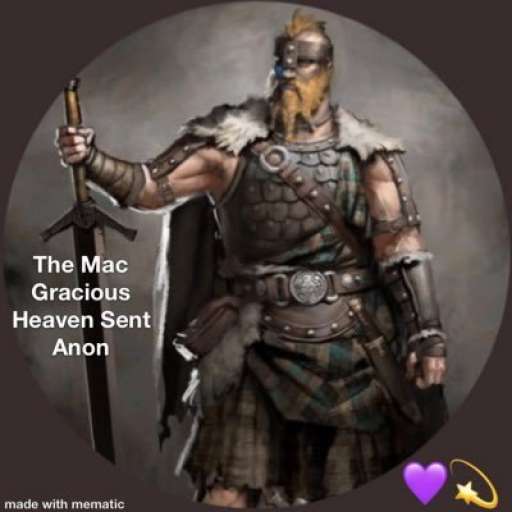
The Sagnac effect manifests itself in a setup called a ring interferometer. A beam of light is split and the two beams are made to follow the same path but in opposite directions. On return to the point of entry the two light beams are allowed to exit the ring and undergo interference.