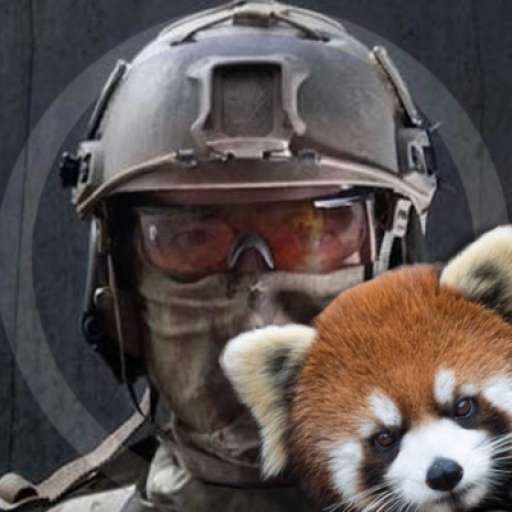
#FamilyIsEverything. APL = 5.55/10 *Authorized conspiracy theorist *Not an authorized preacher.
Cuz just went to the Hospital. He was avoiding it for a week. Took all the right meds. High does of ivermectin, zinc, quercetin, Vit D. Oxegen. AntiVax household. Hate that he is in there right now. Strict orders on No Vent or Remdes. Aunt is a vaxxer and shaming them for not getting the Vax. What a web.

QSI Telegram, learning about Quantum Stellar Financial System, Medbeds, Liberty/Freedom, Checks and Balances, disclosure, Ascension,💜♾️
Auto immune issues, the body having nanobots manipulating and controlling, causing imbalance?
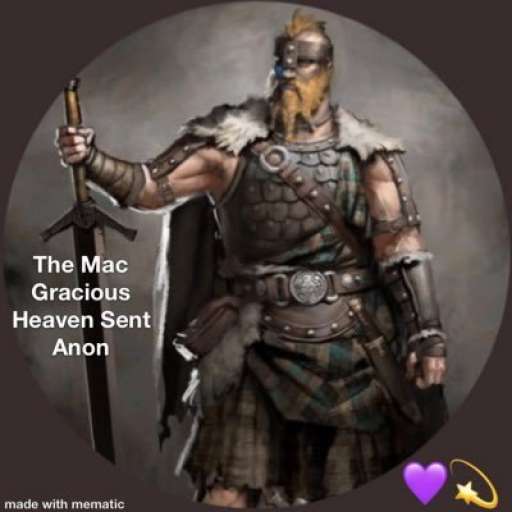
Or the immune sytem cannot make a distinction between the body’s own fatty lipid cell membranes compared to the fatty acid lipids that come from fatty lipid membranes that encapsulate solid lipid nanoparticle vaccines?
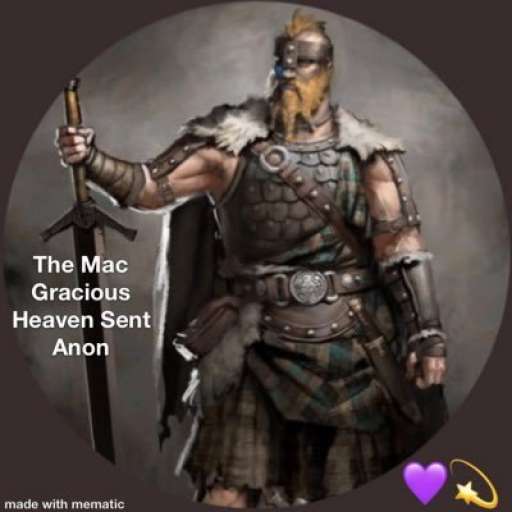
Fusion is the process by which two lipid bilayers merge, resulting in one connected structure. If this fusion proceeds completely through both leaflets of both bilayers, a water-filled bridge is formed and the solutions contained by the bilayers can mix. Alternatively, if only one leaflet from each bilayer is involved in the fusion process, the bilayers are said to be hemifused. Fusion is involved in many cellular processes, in particular in eukaryotes, since the eukaryotic cell is extensively sub-divided by lipid bilayer membranes. Exocytosis, fertilization of an egg by sperm activation, and transport of waste products to the lysozome are a few of the many eukaryotic processes that rely on some form of fusion. Even the entry of pathogens can be governed by fusion, as many bilayer-coated viruses have dedicated fusion proteins to gain entry into the host cell.

QSI Telegram, learning about Quantum Stellar Financial System, Medbeds, Liberty/Freedom, Checks and Balances, disclosure, Ascension,💜♾️
Absolutely love the information shared on the mac page.
Freshing and unique, Thank Q, blissing with information, creating fusion?
Viruses Or nanoparticles acting as viruses?
What kind, type of viruses?
What viruses are concerns for humans?
Where do viruses come from?
Are there viruses from nature?
Are viruses man made?
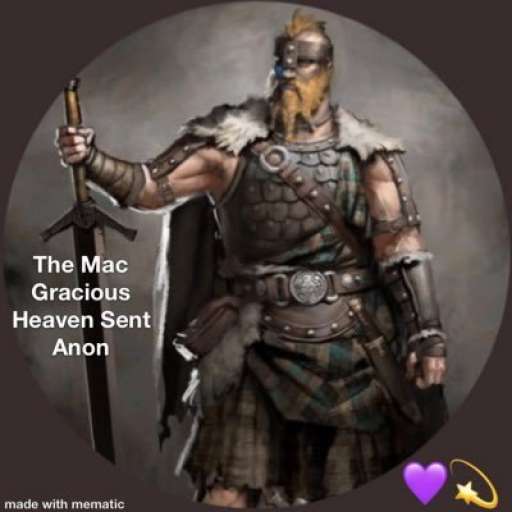
Tobacco mosaic virus was the first virus to be crystallized. It was achieved by Wendell Meredith Stanley in 1935 who also showed that TMV remains active even after crystallization.
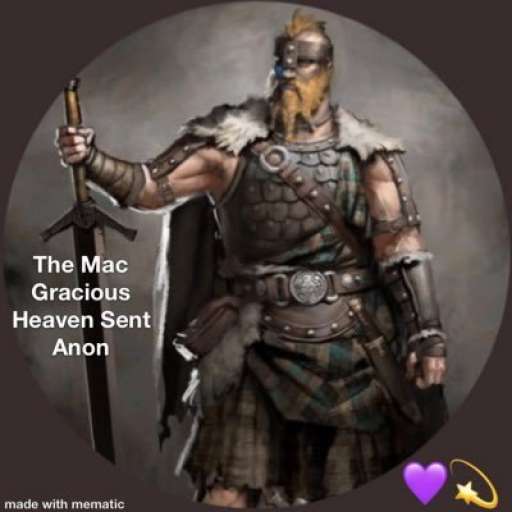
The positive influence of ultrasound (US) on crystallization processes is shown by the dramatic reduction of the induction period, supersaturation conditions and metastable zone width. Manipulation of this influence can be achieved by changing US-related variables such as frequency, intensity, power and even geometrical characteristics of the ultrasonic device (e.g. horn type size). The volume of the sonicated solution and irradiation time are also variables to be optimized in a case-by-case basis as the mechanisms of US action on crystallization remain to be established. Nevertheless, the results obtained so far make foreseeable that crystal size distribution, and even crystal shape, can be ‘tailored’ by appropriate selection of the sonication conditions.
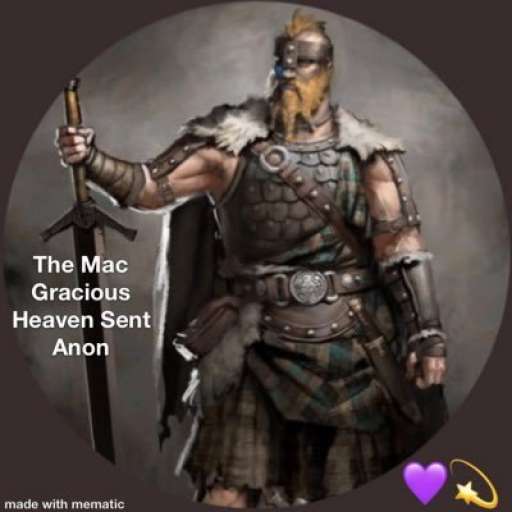
For semiconductors, doping is an efficient approach to tune their energy bandgaps, charge transport, and optical properties which could enable the improvement of the corresponding performances and open up the possibility of multifunction integration. Recently, significant advances have been achieved in molecular doped organic semiconductors, especially doped organic semiconductor single crystals (OSSCs) which have features of well-defined packing structures, long-range molecular orders, and low-density defects for fundamental studies and improved properties. In this review, we will give a summary of the exciting progress of molecular doped OSSCs from the aspects of selection criteria of molecular dopants, general growth methods, and resulting optoelectronic properties as well as their applications in optoelectronic devices. Finally, a brief conclusion is given with challenges and perspectives of molecular doped OSSCs and their related promising research directions in this field.
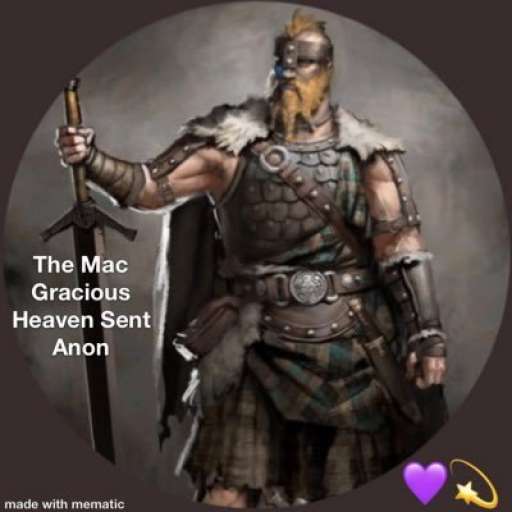
Crystalline silicon is the dominant semiconducting material used in photovoltaic technology for the production of solar cells. These cells are assembled into solar panels as part of a photovoltaic system to generate solar power from sunlight.
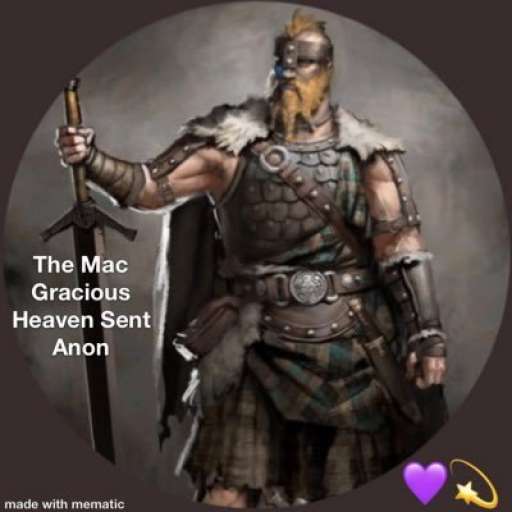
A photodiode is a semiconductor p–n junction device that converts light into an electrical current.[1] The current is generated when photons are absorbed in the photodiode. Photodiodes may contain optical filters, built-in lenses, and may have large or small surface areas. Photodiodes usually have a slower response time as their surface area increases. The common, traditional solar cell used to generate electric solar power is a large area photodiode.
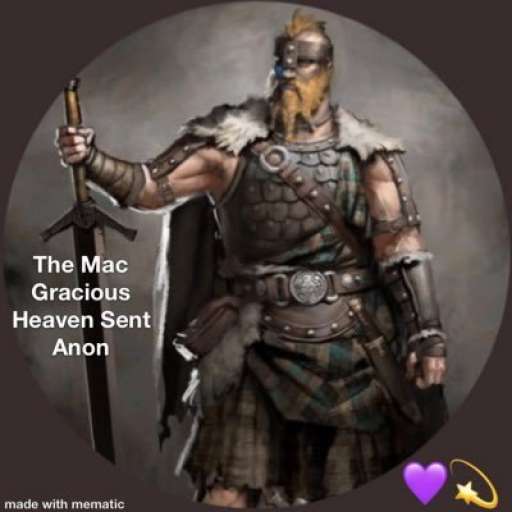
Solid-solution organic crystals have been brought into the quest for superior photon upconversion materials, which transform presently wasted long-wavelength light into more useful shorter wavelength light. Scientists revisited a materials approach previously deemed lackluster -- using a molecule originally developed for organic LEDs -- achieving outstanding performance and efficiency. Their findings pave the way for many novel photonic technologies, such as better solar cells and photocatalysts for hydrogen and hydrocarbon productions.
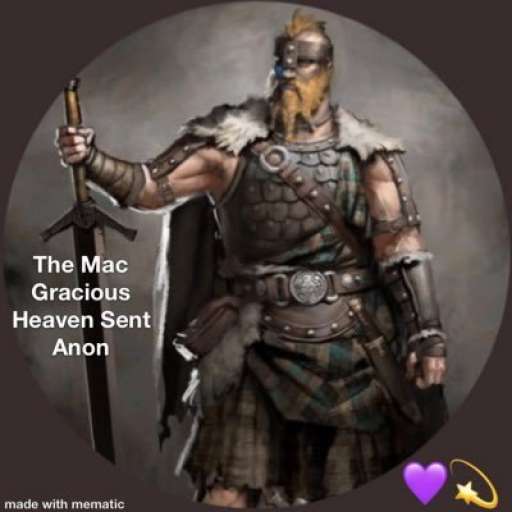
A photonic crystal is an optical nanostructure in which the refractive index changes periodically. This affects the propagation of light in the same way that the structure of natural crystals gives rise to X-ray diffraction and that the atomic lattices of semiconductors affect their conductivity of electrons.
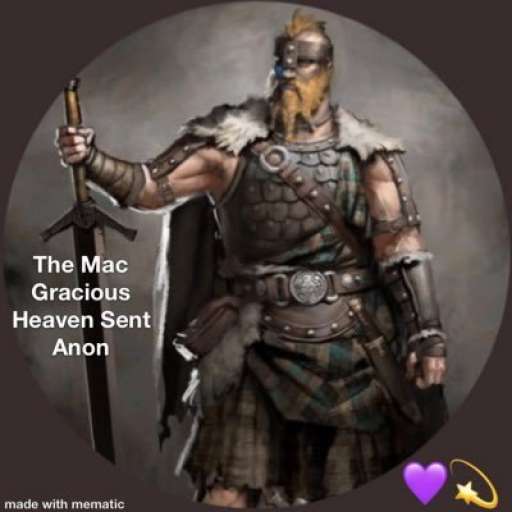
Magnetite is a mineral and one of the main iron ores, with the chemical formula Fe3O4. It is one of the oxides of iron, and is ferrimagnetic; it is attracted to a magnet and can be magnetized to become a permanent magnet itself. It is the most magnetic of all the naturally occurring minerals on Earth. Naturally magnetized pieces of magnetite, called lodestone, will attract small pieces of iron, which is how ancient peoples first discovered the property of magnetism.
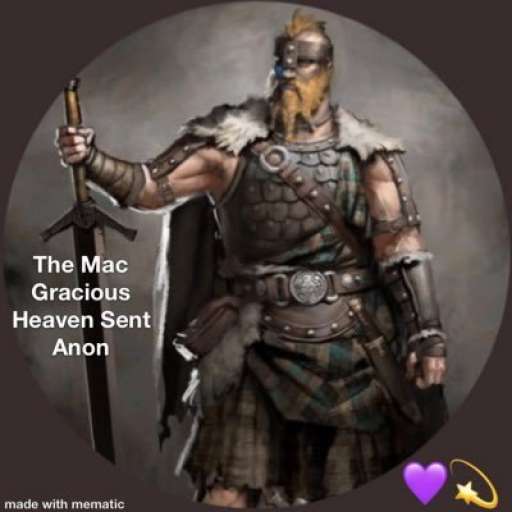
Natural and synthetic magnetite occurs most commonly as octahedral crystals bounded by planes and as rhombic-dodecahedra. Twinning occurs on the plane.
Hydrothermal synthesis usually produces single octahedral crystals which can be as large as 10 mm (0.39 in) across. In the presence of mineralizers such as 0.1 M HI or 2 M NH4Cl and at 0.207 MPa at 416–800 °C, magnetite grew as crystals whose shapes were a combination of rhombic-dodechahedra forms. The crystals were more rounded than usual. The appearance of higher forms was considered as a result from a decrease in the surface energies caused by the lower surface to volume ratio in the rounded crystals.[
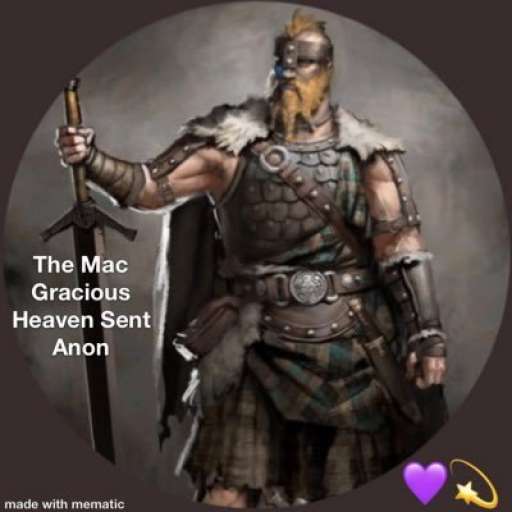
Crystal structure
The chemical composition of magnetite is Fe2+(Fe3+)2(O2-)4. This indicates that magnetite contains both ferrous (divalent) and ferric (trivalent) iron, suggesting crystallization in an environment containing intermediate levels of oxygen. The main details of its structure were established in 1915. It was one of the first crystal structures to be obtained using X-ray diffraction. The structure is inverse spinel, with O2− ions forming a face-centered cubic lattice and iron cations occupying interstitial sites. Half of the Fe3+ cations occupy tetrahedral sites while the other half, along with Fe2+ cations, occupy octahedral sites. The unit cell consists of 32 O2− ions and unit cell length is a = 0.839 nm.
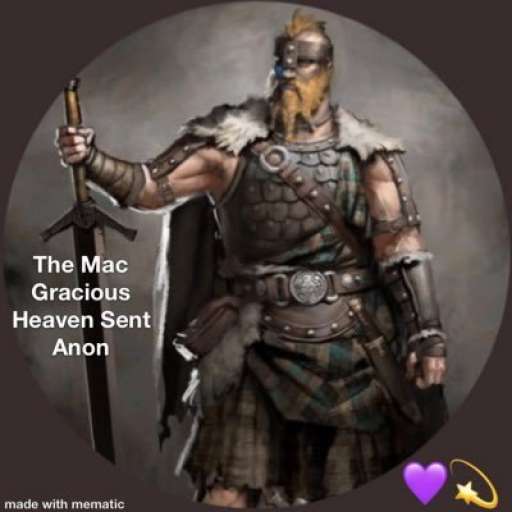
Biomagnetism is usually related to the presence of biogenic crystals of magnetite, which occur widely in organisms. These organisms range from magnetotactic bacteria (e.g., Magnetospirillum magnetotacticum) to animals, including humans, where magnetite crystals (and other magnetically sensitive compounds) are found in different organs, depending on the species. Biomagnetites account for the effects of weak magnetic fields on biological systems. There is also a chemical basis for cellular sensitivity to electric and magnetic fields (galvanotaxis).
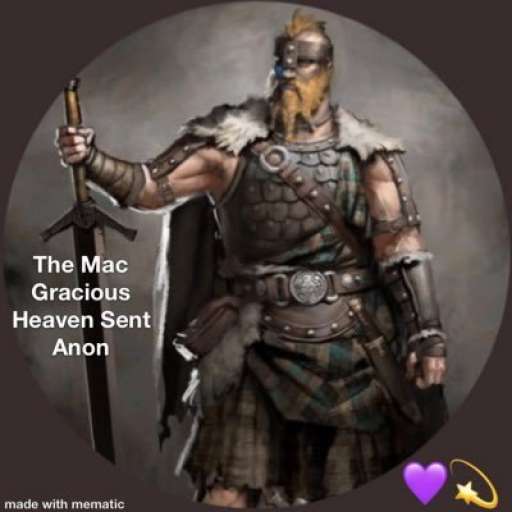
Pure magnetite particles are biomineralized in magnetosomes, which are produced by several species of magnetotactic bacteria. Magnetosomes consist of long chains of oriented magnetite particle that are used by bacteria for navigation. After the death of these bacteria, the magnetite particles in magnetosomes may be preserved in sediments as magnetofossils. Some types of anaerobic bacteria that are not magnetotactic can also create magnetite in oxygen free sediments by reducing amorphic ferric oxide to magnetite.
Several species of birds are known to incorporate magnetite crystals in the upper beak for magnetoreception, which (in conjunction with cryptochromes in the retina) gives them the ability to sense the direction, polarity, and magnitude of the ambient magnetic field.
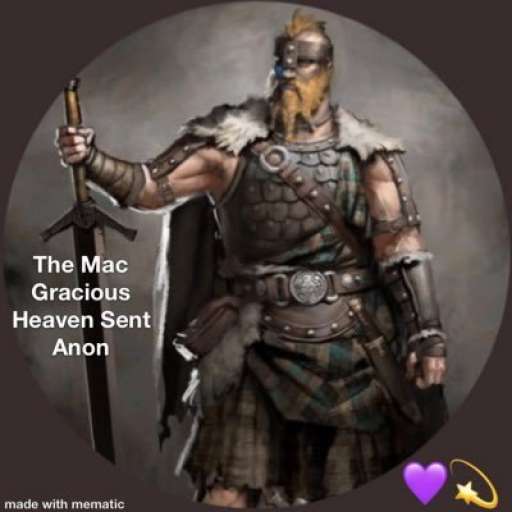
Living organisms can produce magnetite. In humans, magnetite can be found in various parts of the brain including the frontal, parietal, occipital, and temporal lobes, brainstem, cerebellum and basal ganglia. Iron can be found in three forms in the brain – magnetite, hemoglobin (blood) and ferritin (protein), and areas of the brain related to motor function generally contain more iron. Magnetite can be found in the hippocampus. The hippocampus is associated with information processing, specifically learning and memory. However, magnetite can have toxic effects due to its charge or magnetic nature and its involvement in oxidative stress or the production of free radicals. Research suggests that beta-amyloid plaques and tau proteins associated with neurodegenerative disease frequently occur after oxidative stress and the build-up of iron.
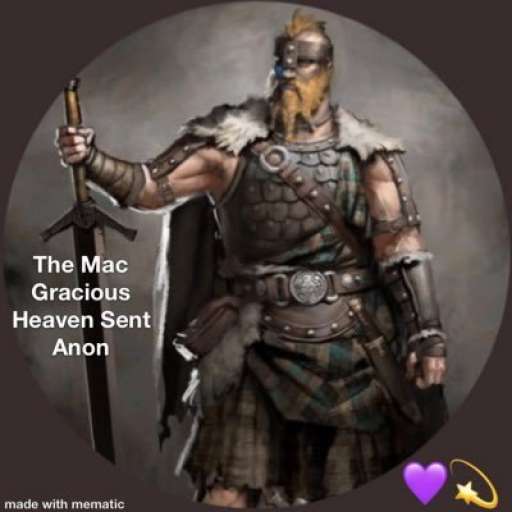
Iron oxide nanoparticles are iron oxide particles with diameters between about 1 and 100 nanometers. The two main forms are magnetite (Fe3O4) and its oxidized form maghemite (γ-Fe2O3). They have attracted extensive interest due to their superparamagnetic properties and their potential applications in many fields (although cobalt and nickel are also highly magnetic materials, they are toxic and easily oxidized).
Applications of iron oxide nanoparticles include terabit magnetic storage devices, catalysis, sensors, superparamagnetic relaxometry, high-sensitivity biomolecular magnetic resonance imaging, magnetic particle imaging, magnetic fluid hyperthermia, separation of biomolecules, and targeted drug and gene delivery for medical diagnosis and therapeutics. These applications require coating of the nanoparticles by agents such as long-chain fatty acids, alkyl-substituted amines, and diols.[citation needed] They have been used in formulations for supplementation.
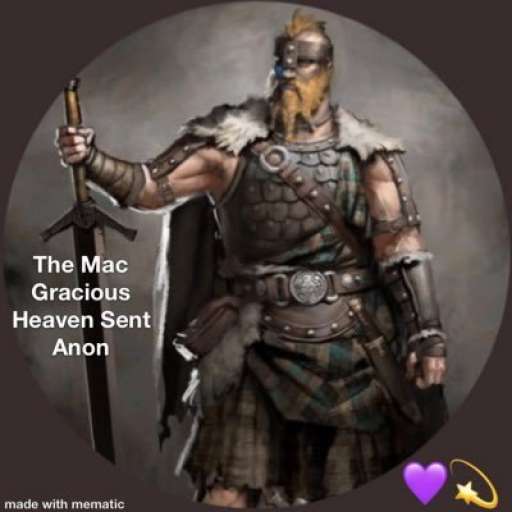
Long chain fatty acids (LCFA), frequently called free fatty acids or nonesterified fatty acids, are straight chain fatty acids containing twelve or more carbon atoms. Because LCFA are usually synthesized in animals or plants from acetyl-CoA and are then degraded two carbons at a time via β-oxidation in animals, the LCFA found most commonly in animals have an even number of carbon atoms.
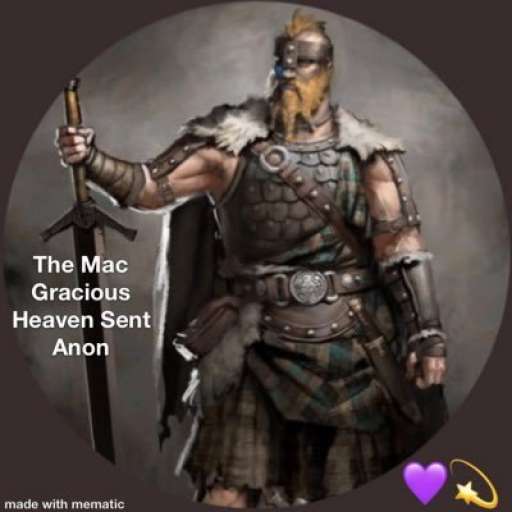
LCFA having carbon chain lengths of 16 and 18 constitute the greatest bulk of fatty acids in animal tissues and most animal diets. The saturated 16-carbon LCFA is palmitic acid, and the saturated 18-carbon LCFA is stearic acid. Unsaturated 18-carbon LCFA are common, with double bonds occurring at C9—C10 (oleic acid); at C9—C10 and C12—C13 (linoleic acid); and at C9—C10, C12—C13, and C15—C16 (linolenic acid). The double bonds found in fatty acids in nature are mostly of the cis configuration. Ruminant fat contains more trans-LCFA than that of nonruminants because rumen microbes isomerize some plant cis-LCFA to trans isomers.
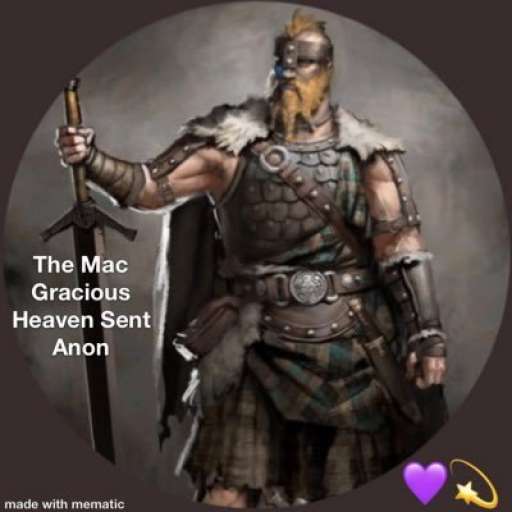
Unsaturated LCFA have a lower melting point than saturated LCFA with the same number of carbons and are more susceptible to spontaneous oxidation (Gurr et al., 2002). The 20-carbon polyunsaturated fatty acids, arachidonic acid (double bonds at C5—C6, C8—C9, C11—C12, C14—C15) and eicosapentaenoic acid (also called timnodonic acid), which is arachidonic acid with an additional double bond at C17—C18, are the precursors of the eicosanoids (prostaglandins, leukotrienes, thromboxanes).
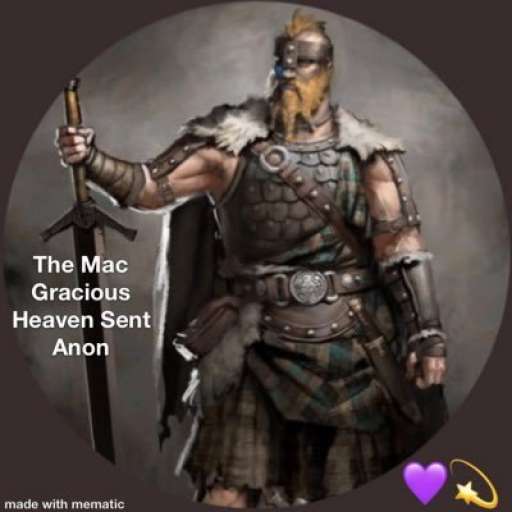
Long chain fatty acids are relatively insoluble in water at physiological pH. They dissolve readily in highly alkaline solutions, forming soaps. LCFA are amphiphilic, being quite polar (hydrophilic) at their carboxyl end and quite nonpolar (hydrophobic) at the methyl end. All LCFA must bind to proteins in order to be transported for any significant distance, and albumin is the primary transport protein in plasma (Gurr et al., 2002).
Plasma LCFA concentrations can be determined spectrophotometrically with a specific enzymatic reaction, which involves direct reaction of plasma LCFA to form LCFA-CoA.
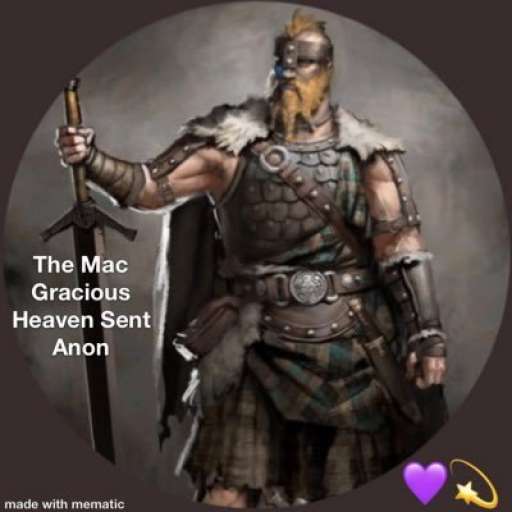
Then, LCFA-CoA is oxidized using LCFA-CoA oxidase, which produces hydrogen peroxide. The hydrogen peroxide is used to produce a colored product under the catalysis of peroxidase (Demacker et al., 1982; Shimizu et al., 1980). If a sample contains triacylglycerol and lipase, which is not uncommon, LCFA may be released if the sample is allowed to stand. Falsely high LCFA may be avoided by centrifuging blood samples and freezing the plasma immediately after collection or by adding paraoxon, a lipase inhibitor (Degen and Van der Vies, 1985).
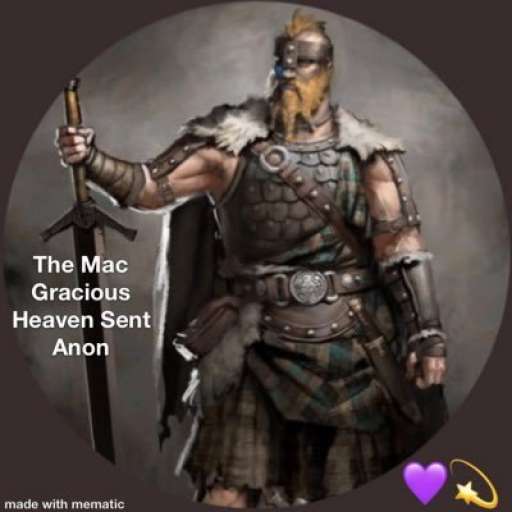
Long-Chain Polyunsaturated Fatty Acids.
Long-chain polyunsaturated fatty acids (LCPUFAs) are usually thought of as structural components of cell membranes, but they also function in a number of intracellular signaling and metabolic pathways involved in the pathogenesis of vasoproliferative and retinal neurodegenerative disorders.199
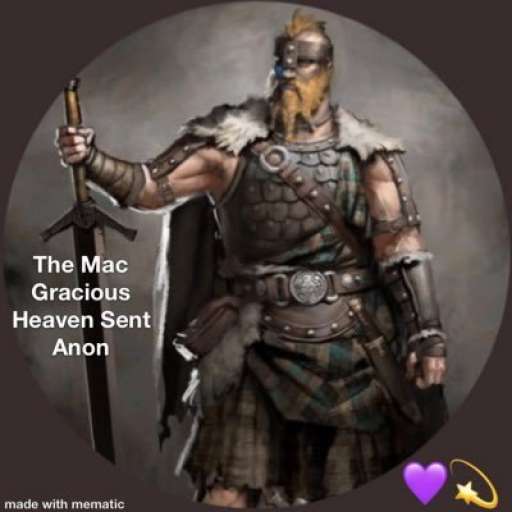
Dietary omega-3 LCPUFAs are important in normal retinal function as well as neuroprotection. Docosahexaenoic acid (DHA) in particular is important for photoreceptor metabolism and transduction mechanisms and it makes up the largest fraction of the LCPUFAs in the outer-segment disc membranes.200 Lowered dietary intake of LCPUFAs reduced susceptibility of the rat retina to light-induced photoreceptor damage,201 possibly through a slowing of the regeneration rate of rhodopsin.202
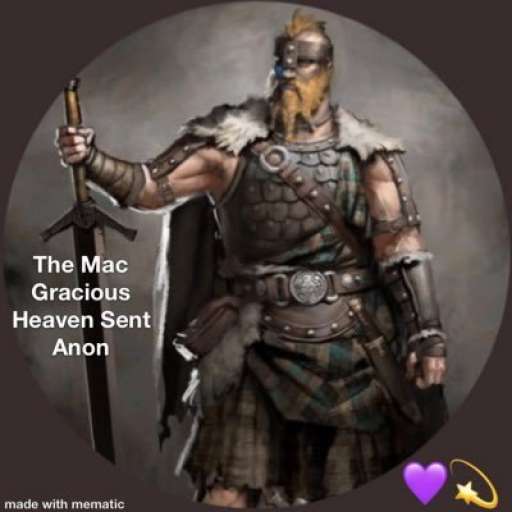
Dietary effects on the levels of retinal LCPUFAs may affect a variety of retinal molecules and signaling pathways involved in neuroprotection, including dopamine, cyclic nucleotides, endocannabinoids, glutamate, and ionic transport and channel dynamics.199 Metabolic and environmental activators of LCPUFAs, phospholipase A2, cyclooxygenase, and lipoxygenase are associated with a number of retinal diseases, including diabetic retinopathy, AMD, and retinopathy of prematurity through modulation of neuronal and vascular function.199 A major dietary source of LCPUFAs is fish. Incidence of AMD was shown to decrease with increasing intake of DHA, tuna, or total fish.203 Several other studies have shown similar trends,199 but the neuroprotective mechanism is not known.
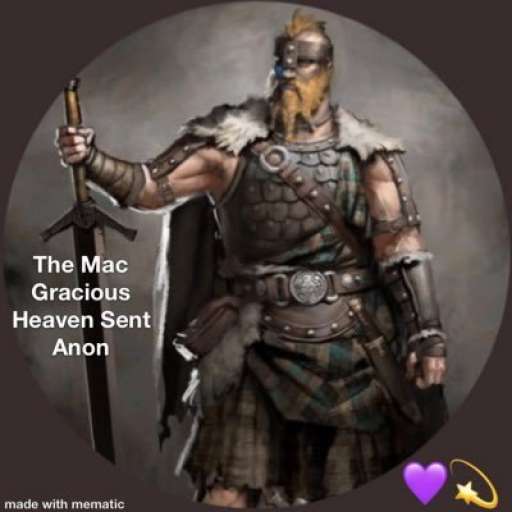
The natural recycling of membrane phospholipids produces neuroprotectin D1 (NPD1), the first known bioactive product of DHA. This molecule is produced by the action of phospholipase A2 that releases free DHA from membrane phospholipids under conditions of oxidative stress and inflammation and promotes cell survival in animal models of stroke and Alzheimer's disease204,205 and protects RPE cells from oxidative stress.206,207 The latter effect is linked to activation of the phosphatidylinositol 3-kinase/Akt signaling pathway through increased phosphorylation.206 Though a direct effect on photoreceptor neuroprotection has so far not been demonstrated, NPD1 produced in RPE upon daily phagocytosis of photoreceptor membranous discs, which contain large amounts of esterified DHA, may be released and promote the survival of other retinal cells in a paracrine fashion.208
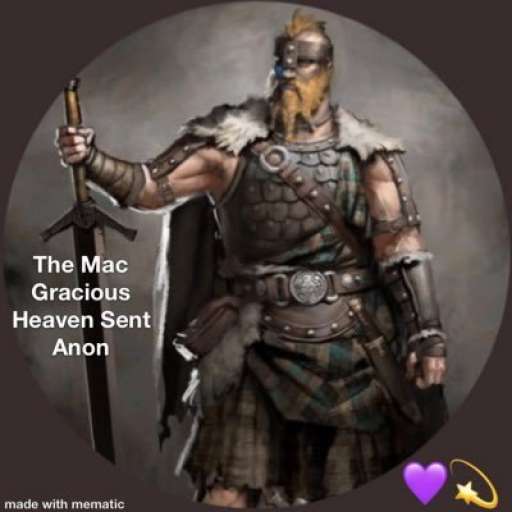
Rhodopsin is a G-protein coupled receptor found in the rod cells of the retina. As a biomarker associated with retinal thinning and degeneration, it bears potential in the early detection and monitoring of several neurodegenerative diseases.
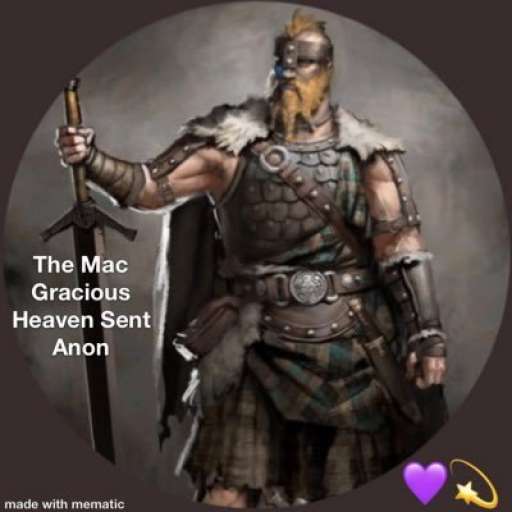
Channelrhodopsins are a subfamily of retinylidene proteins (rhodopsins) that function as light-gated ion channels. They serve as sensory photoreceptors in unicellular green algae, controlling phototaxis: movement in response to light. Expressed in cells of other organisms, they enable light to control electrical excitability, intracellular acidity, calcium influx, and other cellular processes (see optogenetics). Channelrhodopsin-1 (ChR1) and Channelrhodopsin-2 (ChR2) from the model organism Chlamydomonas reinhardtii are the first discovered channelrhodopsins. Variants have been cloned from other algal species, and more are expected.
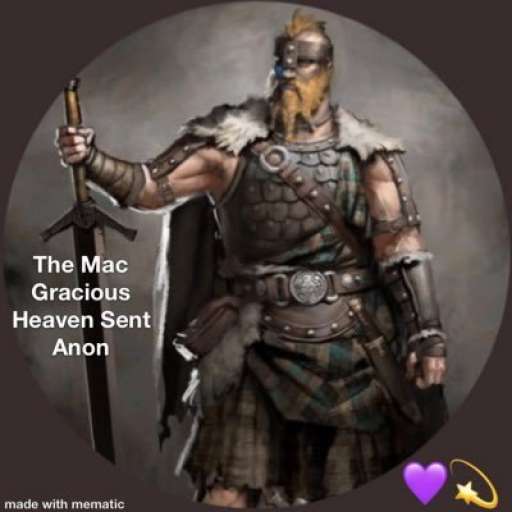
Current neuromodulation techniques such as optogenetics and deep-brain stimulation are transforming basic and translational neuroscience. These two neuromodulation approaches are, however, invasive since surgical implantation of an optical fiber or wire electrode is required. Here, we have invented a non-invasive magnetogenetics that combines the genetic targeting of a magnetoreceptor with remote magnetic stimulation.
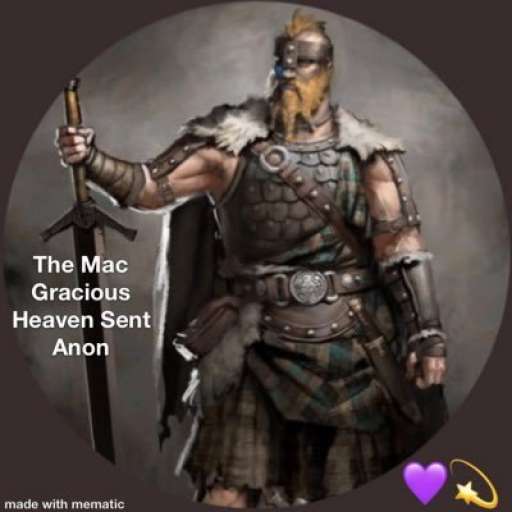
The non-invasive activation of neurons was achieved by neuronal expression of an exogenous magnetoreceptor, an iron-sulfur cluster assembly protein 1 (Isca1). In HEK-293 cells and cultured hippocampal neurons expressing this magnetoreceptor, application of an external magnetic field resulted in membrane depolarization and calcium influx in a reproducible and reversible manner, as indicated by the ultrasensitive fluorescent calcium indicator GCaMP6s.
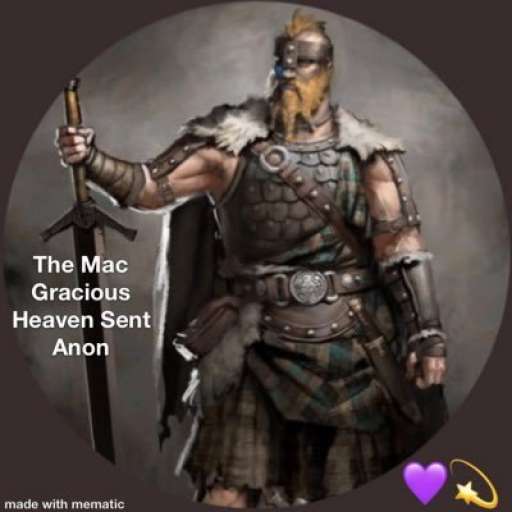
Moreover, the magnetogenetic control of neuronal activity might be dependent on the direction of the magnetic field and exhibits on-response and off-response patterns for the external magnetic field applied. The activation of this magnetoreceptor can depolarize neurons and elicit trains of action potentials, which can be triggered repetitively with a remote magnetic field in whole-cell patch-clamp recording. In transgenic Caenorhabditis elegans expressing this magnetoreceptor in myo-3-specific muscle cells or mec-4-specific neurons, application of the external magnetic field triggered muscle contraction and withdrawal behavior of the worms, indicative of magnet-dependent activation of muscle cells and touch receptor neurons, respectively.
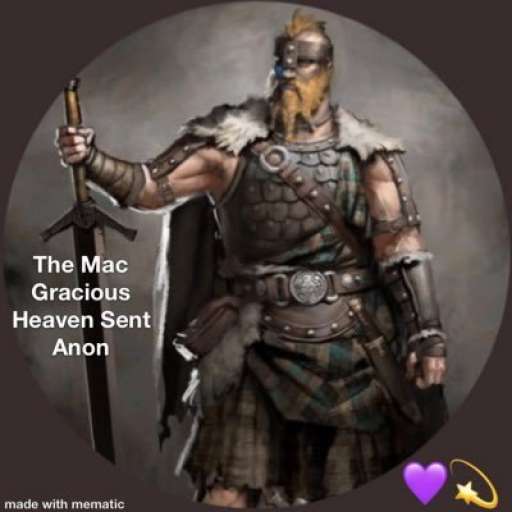
The advantages of magnetogenetics over optogenetics are its exclusive non-invasive, deep penetration, long-term continuous dosing, unlimited accessibility, spatial uniformity and relative safety. Like optogenetics that has gone through decade-long improvements, magnetogenetics, with continuous modification and maturation, will reshape the current landscape of neuromodulation toolboxes and will have a broad range of applications to basic and translational neuroscience as well as other biological sciences. We envision a new age of magnetogenetics is coming.
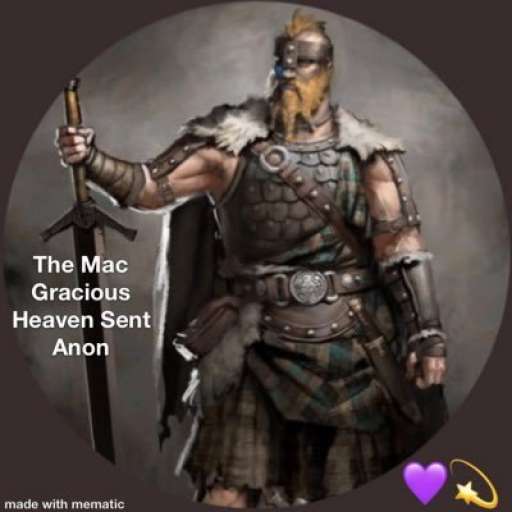
Sonogenetics, which uses ultrasound to noninvasively control cells genetically modified with ultrasound-sensitive ion channels, can be a powerful tool for investigating intact brain circuits. Here we show that TRPV1 is an ultrasound-sensitive ion channel that can modify the activity of TRPV1-expressing cells in vitro when exposing to focused ultrasound. We also show that focused ultrasound exposure at the mouse brain in vivo can selectively activate neurons that are genetically modified to express TRPV1. We demonstrate that precise manipulation of neural activity via TRPV1-based sonogenetics can be achieved by spatiotemporal control of ultrasound-induced heating. The focused ultrasound exposure is safe based on our inspection of neuronal integrity, apoptosis, and inflammation markers. This sonogenetic tool enables noninvasive, cell-type specific, spatiotemporally controlled modulation of mammalian brain activity.
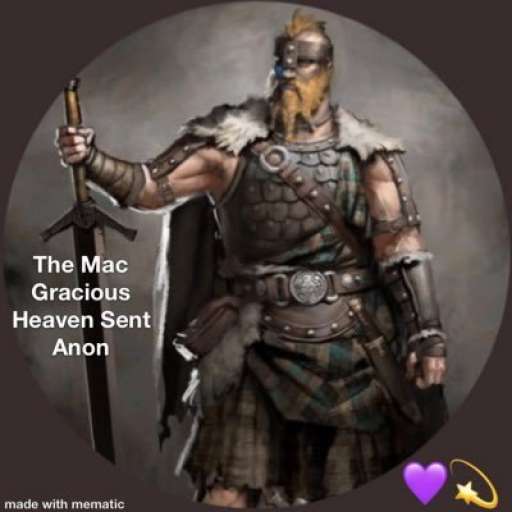
Frontiers | Ultrasonic Neuromodulation and Sonogenetics: A New Era for Neural Modulation | Physiology
Noninvasive ultrasonic neural modulation (UNM), a noninvasive technique with enhanced spatial focus compared to conventional electrical neural modulation, has attracted much attention in recent decades and might become the mainstream regimen for neurological disorders. However, as ultrasonic bioeffe..
https://www.frontiersin.org/articles/10.3389/fphys.2020.00787/full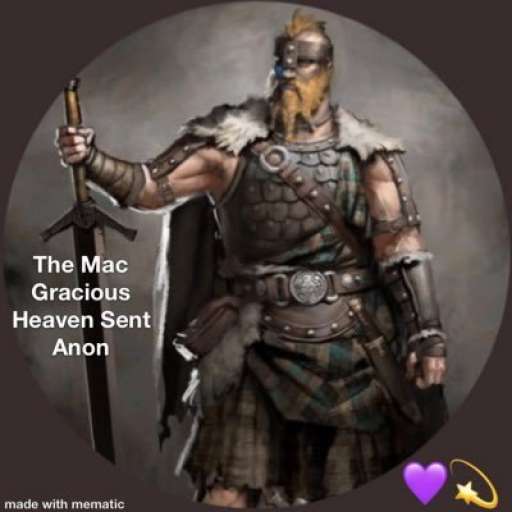
Intensity is the acoustic energy generated by US, usually described as spatial-peak pulse-average intensity (ISPPA) or spatial-peak temporal-average intensity (ISPTA) for application. Intensity is a major determinant of US bioeffects. Based on intensity levels, FUS can be categorized as HIFU or LIFU. HIFU is defined as US with typical intensity levels ranging from approximately 100 W/cm2 to 10000 W/cm2 (Quadri et al., 2018). HIFU was used in early neuromodulation research, while later on, it is more commonly used for ablative surgery to minimize surgical trauma (Klatte et al., 2014).
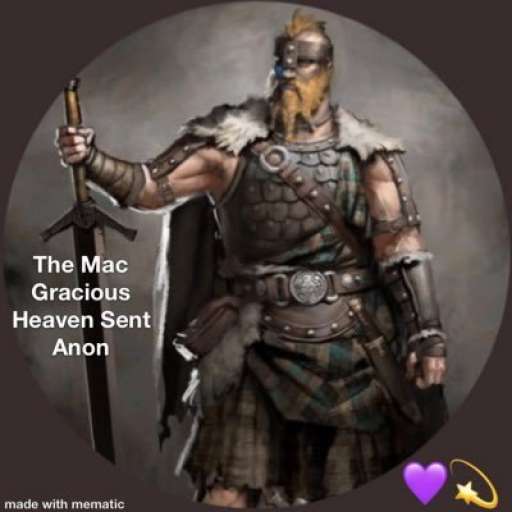
Under high intensity, US exerts bioeffects through thermal elevation and coagulative necrosis, which could lead to irreversible tissue damage. These biological effects were proven to be therapeutic in the surgical treatment of Parkinson’s disease (Strauss et al., 2014) and essential tremor (Dobrakowski et al., 2014; Ahmed et al., 2015). LIFU, with an intensity less than 3 W/m2, could reversibly modulate local tissue under controlled temperatures (Rezayat and Toostani, 2016). This modulatory effect of LIFU is highly intensity dependent. Velling and Shklyaruk (1988) found no detectable effect of LIFU on the brain at low (less than 0.1 mW/cm2) intensities in cats and rabbits. As the intensity increased to 1 to 100 mW/cm2, electrical activity was recorded by US stimulation. However, US with higher intensity from 1 to 100 W/cm2 suppressed brain activity. Therefore, the neural modulatory effect of LIFU is bidirectional, and intensity plays a crucial role.
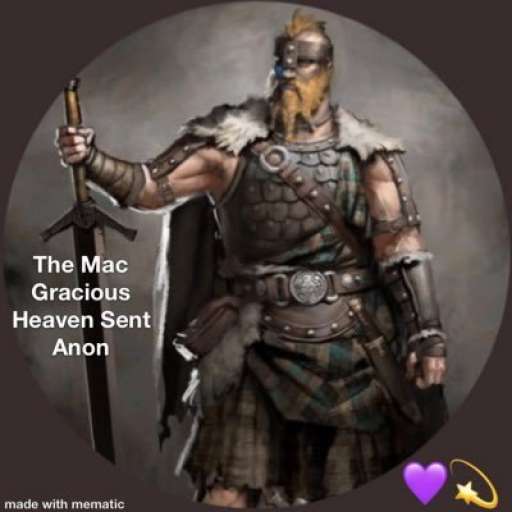
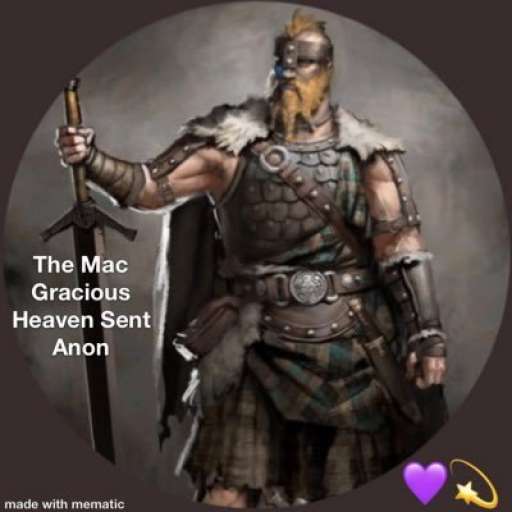
As US propagates in an attenuating pattern due to absorption and scattering, the lost energy subsequently converts to heat.