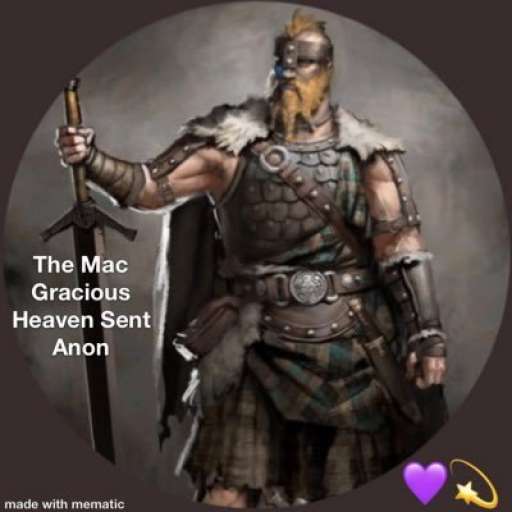
From Middle English gayn, gain, gein (“profit, advantage”), from Old Norse gagn (“benefit, advantage, use”), from Proto-Germanic *gagną, *gaganą (“gain, profit", literally "return”), from Proto-Germanic *gagana (“back, against, in return”), a reduplication of Proto-Germanic *ga- (“with, together”), from Proto-Indo-European *ḱóm (“next to, at, with, along”). Cognate with Icelandic gagn (“gain, advantage, use”), Swedish gagn (“benefit, profit”), Danish gavn (“gain, profit, success”), Gothic 𐌲𐌰𐌲𐌴𐌹𐌲𐌰𐌽 (gageigan, “to gain, profit”), Old Norse gegn (“ready”), dialectal Swedish gen (“useful, noteful”), Latin cum (“with”); see gain-, again, against. Compare also Middle English gaynen, geinen (“to be of use, profit, avail”), Icelandic and Swedish gagna (“to avail, help”), Danish gavne (“to benefit”).
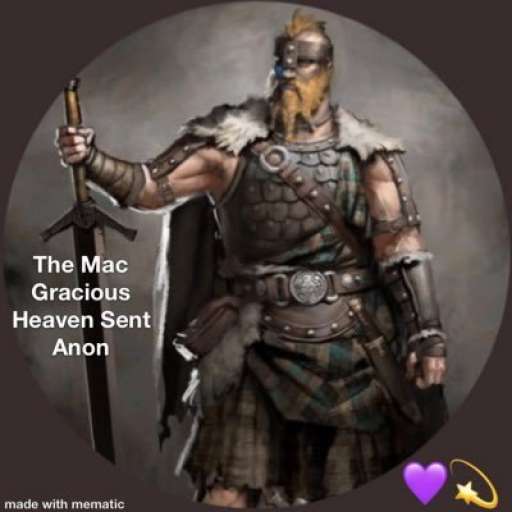
The Middle English word was reinforced by Middle French gain (“gain, profit, advancement, cultivation”), from Old French gaaing, gaaigne, gaigne, a noun derivative of gaaignier (“to till, earn, win”), from Frankish *waidanjan (“to pasture, graze, hunt for food”), ultimately from Proto-Germanic *waiþiz, *waiþō, *waiþijō (“pasture, field, hunting ground”); compare Old High German weidōn, weidanōn (“to hunt, forage for food”) (Modern German Weide (“pasture”)), Old Norse veiða (“to catch, hunt”), Old English wǣþan (“to hunt, chase, pursue”). Related to wathe, wide.
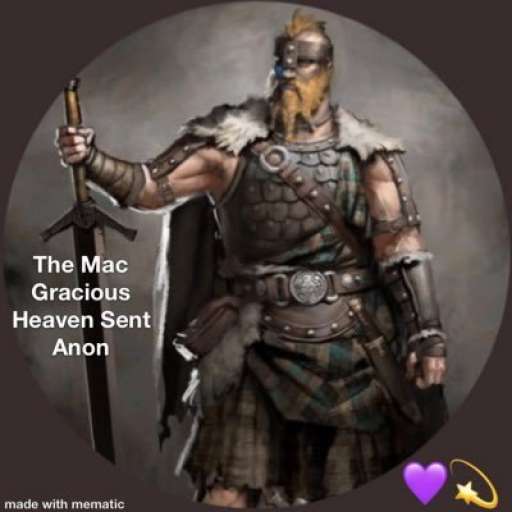
gain (third-person singular simple present gains, present participle gaining, simple past and past participle gained)
(transitive) To acquire possession of.
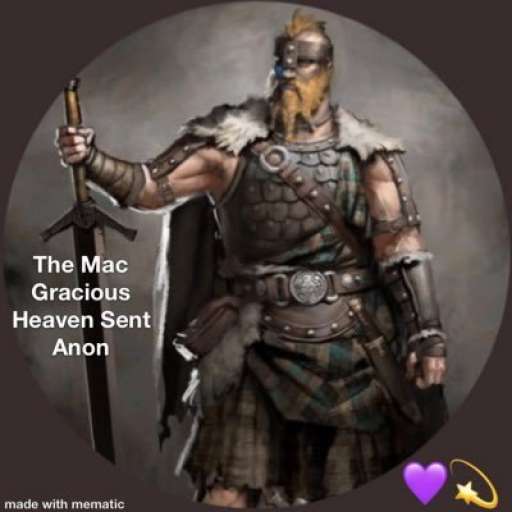
(transitive, dated) To come off winner or victor in; to be successful in; to obtain by competition.
to gain a battle; to gain a case at law
(transitive) To increase.
(intransitive) To be more likely to catch or overtake an individual.
I'm gaining (on you).
gain ground
(transitive) To reach.
to gain the top of a mountain
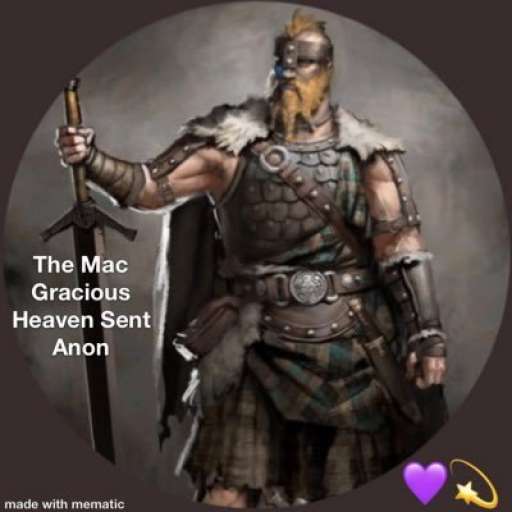
In glaciology, an ice cap is a mass of ice that covers less than 50,000 km2 (19,000 sq mi) of land area (usually covering a highland area). Larger ice masses covering more than 50,000 km2 (19,000 sq mi) are termed ice sheets.
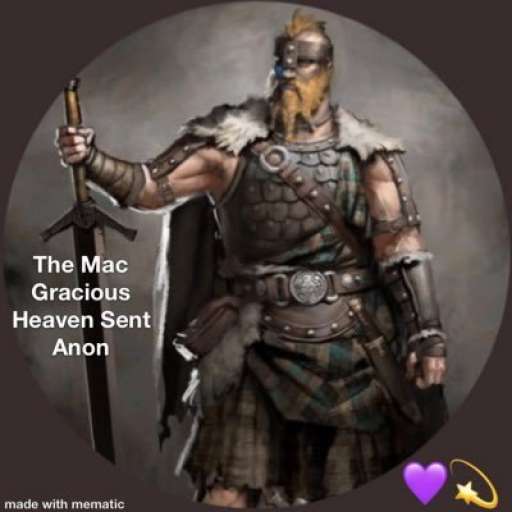
Ice sheets are bigger than ice shelves or alpine glaciers. Masses of ice covering less than 50,000 km2 are termed an ice cap.
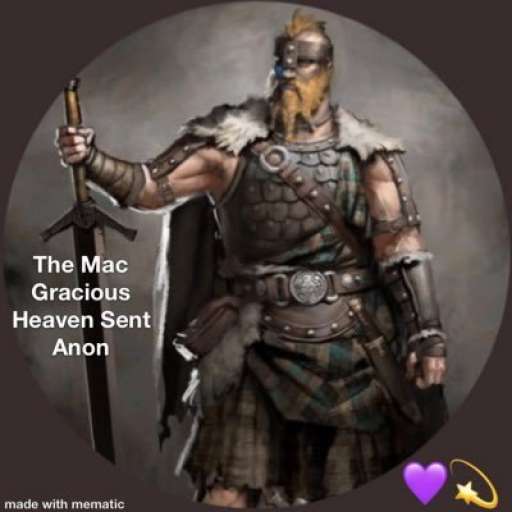
Mass crystallization is the nucleation and growth of a large number of usually small crystals (~10−3–10−1 cm) in one and the same area of space. Examples of it are the formation of metal ingots and kidney stones, the solidification of concrete, and the production of granulated fertilizers, medicines, sugar, and salt.
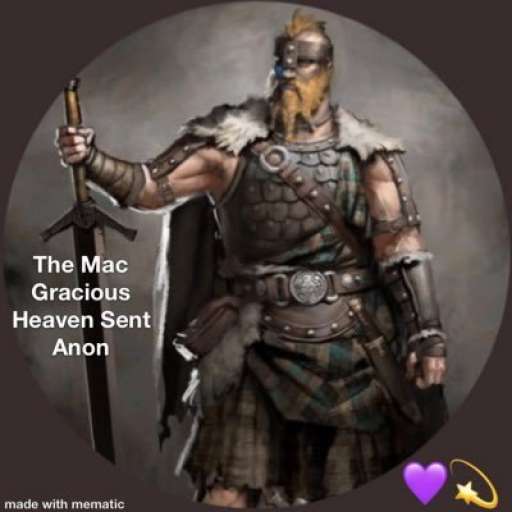
At the present day, ultrafine soot particles have become the object of increasing attention due to their well-documented adverse effects on human health and climate. In particular, understanding soot nucleation is one of the most challenging problems toward a more controlled and cleaner combustion. Detailed information on the chemistry of nascent soot particles (NSPs) is expected to provide clues on the soot formation and growth reaction pathways. Herein, the early steps of soot formation in flames are addressed by investigating the chemical composition of NSPs and their molecular precursors by secondary ion mass spectrometry.
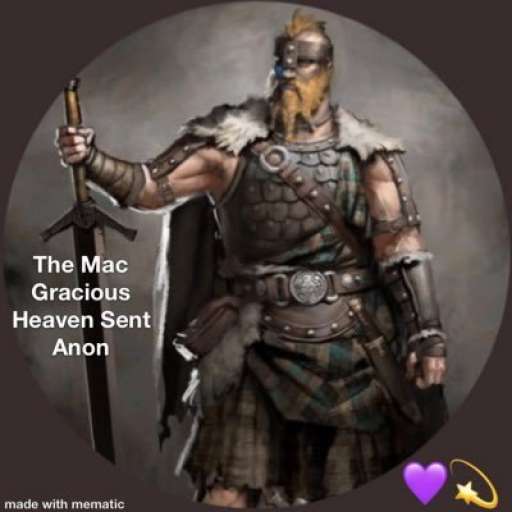
Secondary-ion mass spectrometry (SIMS) is a technique used to analyze the composition of solid surfaces and thin films by sputtering the surface of the specimen with a focused primary ion beam and collecting and analyzing ejected secondary ions. The mass/charge ratios of these secondary ions are measured with a mass spectrometer to determine the elemental, isotopic, or molecular composition of the surface to a depth of 1 to 2 nm. Due to the large variation in ionization probabilities among elements sputtered from different materials, comparison against well-calibrated standards is necessary to achieve accurate quantitative results. SIMS is the most sensitive surface analysis technique, with elemental detection limits ranging from parts per million to parts per billion.
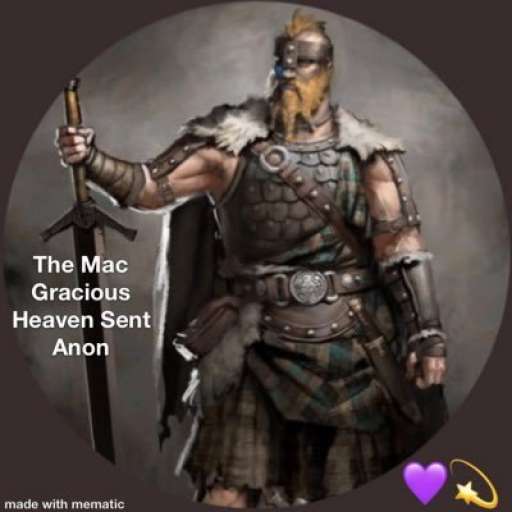
In 1910 British physicist J. J. Thomson observed a release of positive ions and neutral atoms from a solid surface induced by ion bombardment.[1] Improved vacuum pump technology in the 1940s enabled the first prototype experiments on SIMS by Herzog and Viehböck[2] in 1949, at the University of Vienna, Austria.
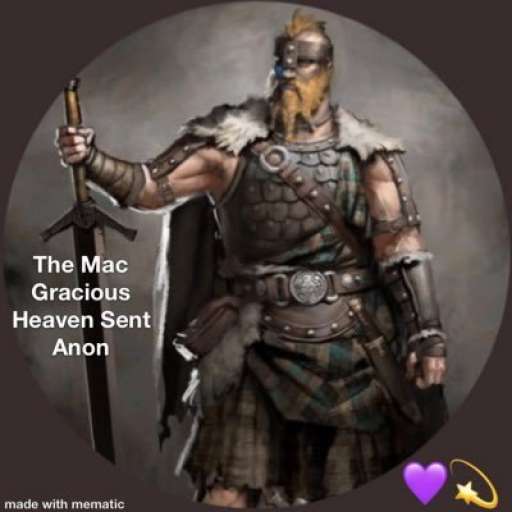
In the mid-1950s Honig constructed a SIMS instrument at RCA Laboratories in Princeton, New Jersey.[3] Then in the early 1960s two SIMS instruments were developed independently.
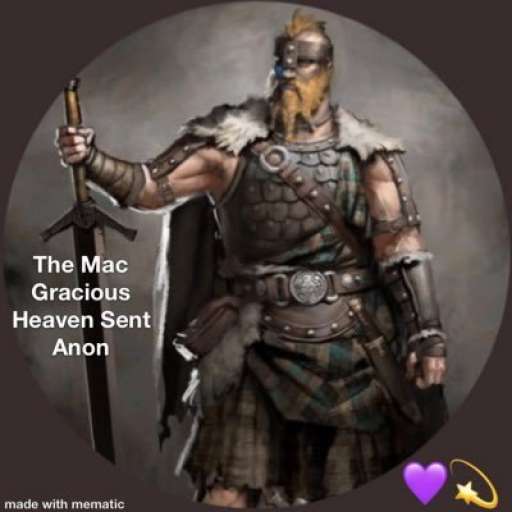
One was an American project, led by Liebel and Herzog, which was sponsored by NASA at GCA Corp, Massachusetts, for analyzing moon rocks,[4] the other at the University of Paris-Sud in Orsay by R. Castaing for the PhD thesis of G. Slodzian.[5] These first instruments were based on a magnetic double focusing sector field mass spectrometer and used argon for the primary beam ions.
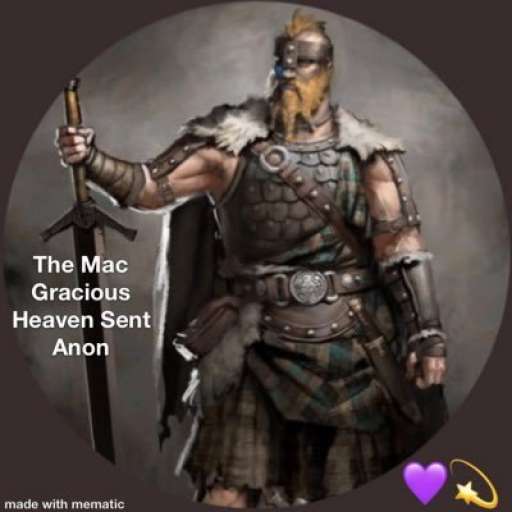
In the 1970s, K. Wittmaack and C. Magee developed SIMS instruments equipped with quadrupole mass analyzers.[6][7] Around the same time, A. Benninghoven introduced the method of static SIMS, where the primary ion current density is so small that only a negligible fraction (typically 1%) of the first surface layer is necessary for surface analysis.[8] Instruments of this type use pulsed primary ion sources and time-of-flight mass spectrometers and were developed by Benninghoven, Niehuis and Steffens at the University of Münster, Germany and also by Charles Evans & Associates.
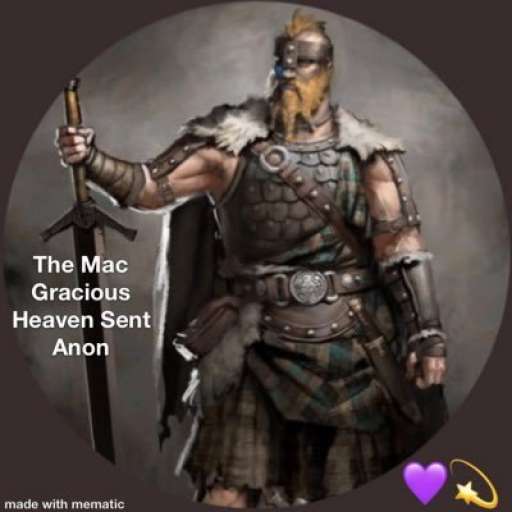
The Castaing and Slodzian design was developed in the 1960s by the French company CAMECA S.A.S. and used in materials science and surface science.[citation needed] Recent developments are focusing on novel primary ion species like C60+, ionized clusters of gold and bismuth,[9] or large gas cluster ion beams (e.g., Ar700+).[10] The sensitive high-resolution ion microprobe (SHRIMP) is a large-diameter, double-focusing SIMS sector instrument based on the Liebl and Herzog design, and produced by Australian Scientific Instruments in Canberra, Australia
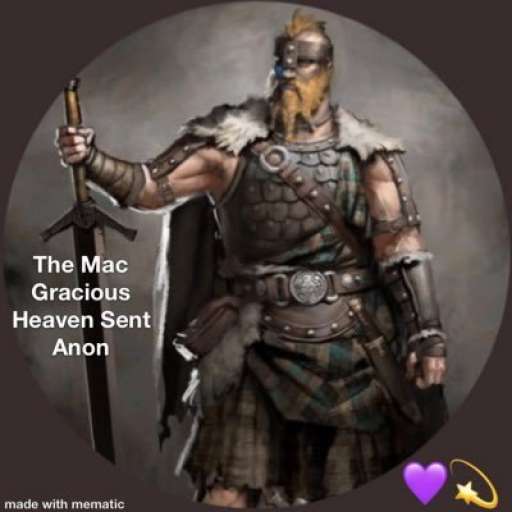
Depending on the SIMS type, there are three basic analyzers available: sector, quadrupole, and time-of-flight. A sector field mass spectrometer uses a combination of an electrostatic analyzer and a magnetic analyzer to separate the secondary ions by their mass-to-charge ratio. A quadrupole mass analyzer separates the masses by resonant electric fields, which allow only the selected masses to pass through. The time of flight mass analyzer separates the ions in a field-free drift path according to their velocity. Since all ions possess the same kinetic energy the velocity and therefore time of flight varies according to mass. It requires pulsed secondary ion generation using either a pulsed primary ion gun or a pulsed secondary ion extraction. It is the only analyzer type able to detect all generated secondary ions simultaneously, and is the standard analyzer for static SIMS instruments.
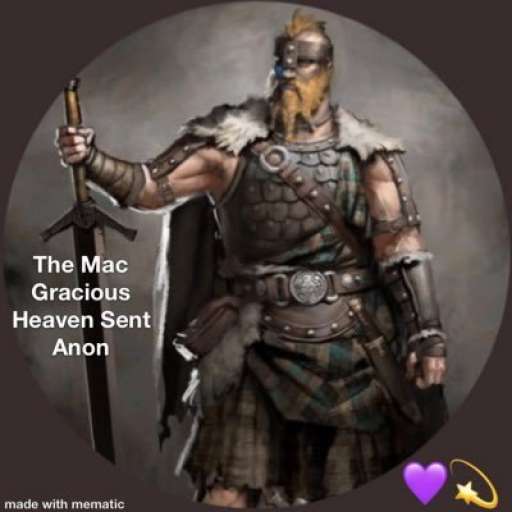
The magnetic sector mass spectrometer causes a physical separation of ions of a different mass-to-charge ratio. The physical separation of the secondary ions is caused by the Lorentz force when the ions pass through a magnetic field that is perpendicular to the velocity vector of the secondary ions. The Lorentz force states that a particle will experience a force
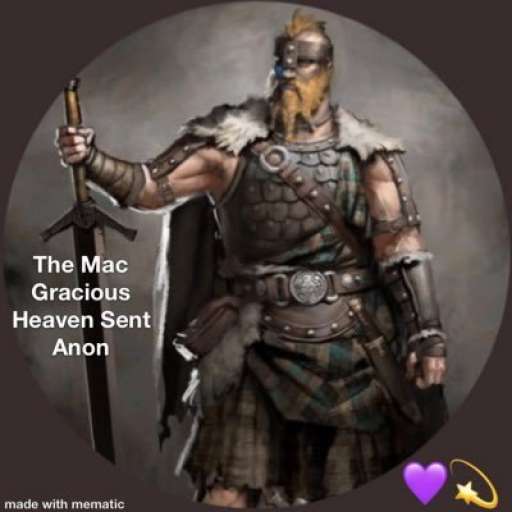
NanoSIMS can capture the spatial variability of isotopic and elemental measurements of sub-micron areas, grains or inclusions from geological, materials science and biological samples.[13] This instrument can characterise nanostructured materials with complex composition that are increasingly important candidates for energy generation and storage.
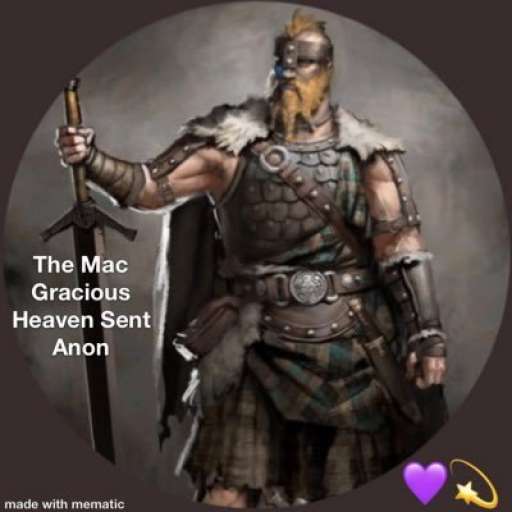
NanoSIMS has also proved useful in studying cosmochemical issues, where samples of single, micro- or sub-micrometer-sized grains from meteorites as well as microtome sections prepared by the focused ion beam (FIB) technique can be analyzed. NanoSIMS can be combined with transmission electron microscopy (TEM) when using microtome or FIB sections. This combination allows for correlated mineralogical and isotopic studies in situ at a sub-micrometer scale.
It is particularly useful in materials research because of its high sensitivity at high mass resolution, which allow for trace element imaging and quantification.[14]
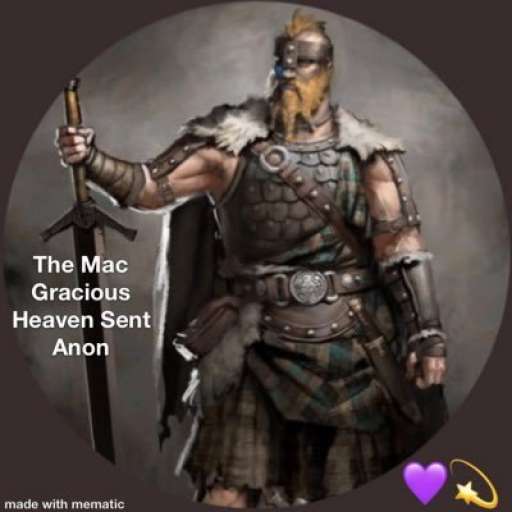
Focused ion beam, also known as FIB, is a technique used particularly in the semiconductor industry, materials science and increasingly in the biological field for site-specific analysis, deposition, and ablation of materials. A FIB setup is a scientific instrument that resembles a scanning electron microscope (SEM). However, while the SEM uses a focused beam of electrons to image the sample in the chamber, a FIB setup uses a focused beam of ions instead. FIB can also be incorporated in a system with both electron and ion beam columns, allowing the same feature to be investigated using either of the beams. FIB should not be confused with using a beam of focused ions for direct write lithography (such as in proton beam writing). These are generally quite different systems where the material is modified by other mechanisms.
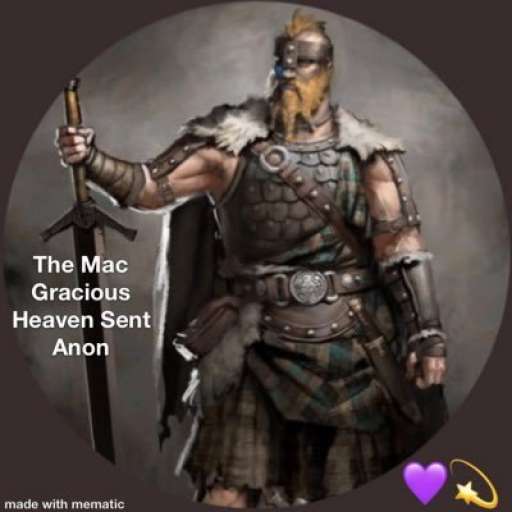
X-ray crystallography is the experimental science determining the atomic and molecular structure of a crystal, in which the crystalline structure causes a beam of incident X-rays to diffract into many specific directions. By measuring the angles and intensities of these diffracted beams, a crystallographer can produce a three-dimensional picture of the density of electrons within the crystal. From this electron density, the mean positions of the atoms in the crystal can be determined, as well as their chemical bonds, their crystallographic disorder, and various other information.
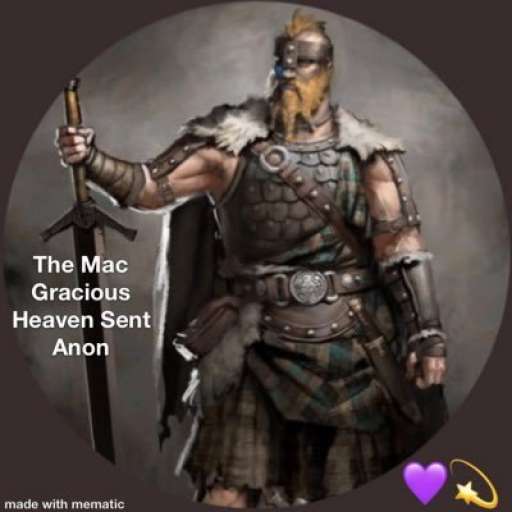
X-ray crystallography of viruses - PubMed
For about 30 years X-ray crystallography has been by far the most powerful approach for determining virus structures at close to atomic resolutions. Information provided by these studies has deeply and extensively enriched and shaped our vision of the virus world. In turn, the ever increasing comple..
https://pubmed.ncbi.nlm.nih.gov/23737050/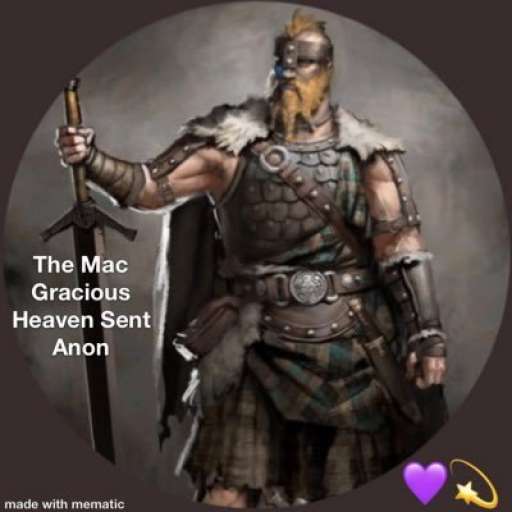
Ultrasound-assisted crystallization (sonocrystallization) - ScienceDirect
The positive influence of ultrasound (US) on crystallization processes is shown by the dramatic reduction of the induction period, supersaturation con…
https://www.sciencedirect.com/science/article/abs/pii/S1350417706001684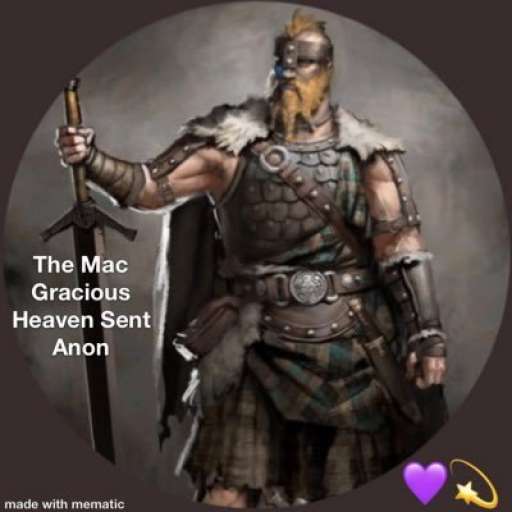
A microprobe is an instrument that applies a stable and well-focused beam of charged particles (electrons or ions) to a sample.
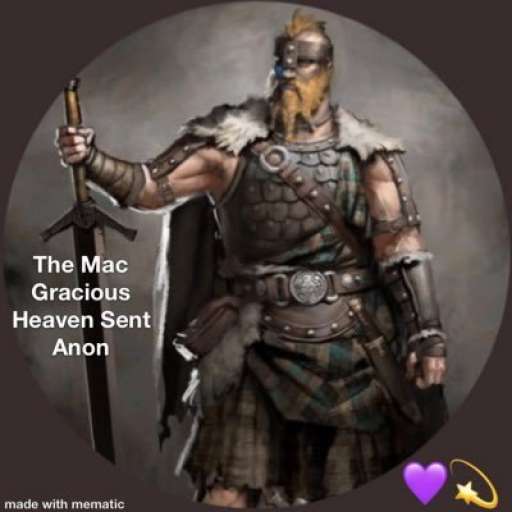
When the primary beam consists of accelerated electrons, the probe is termed an electron microprobe, when the primary beam consists of accelerated ions, the term ion microprobe is used. The term microprobe may also be applied to optical analytical techniques, when the instrument is set up to analyse micro samples or micro areas of larger specimens. Such techniques include micro Raman spectroscopy, micro infrared spectroscopy and micro LIBS. All of these techniques involve modified optical microscopes to locate the area to be analysed, direct the probe beam and collect the analytical signal.
A laser microprobe is a mass spectrometer that uses ionization by a pulsed laser and subsequent mass analysis of the generated ions.
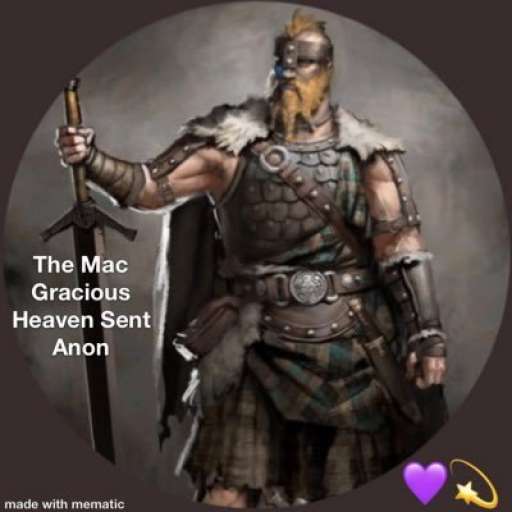
Scientists use this beam of charged particles to determine the elemental composition of solid materials (minerals, glasses, metals).[4] The chemical composition of the target can be found from the elemental data extracted through emitted X-rays (in the case where the primary beam consists of charged electrons) or measurement of an emitted secondary beam of material sputtered from the target (in the case where the primary beam consists of charged ions).
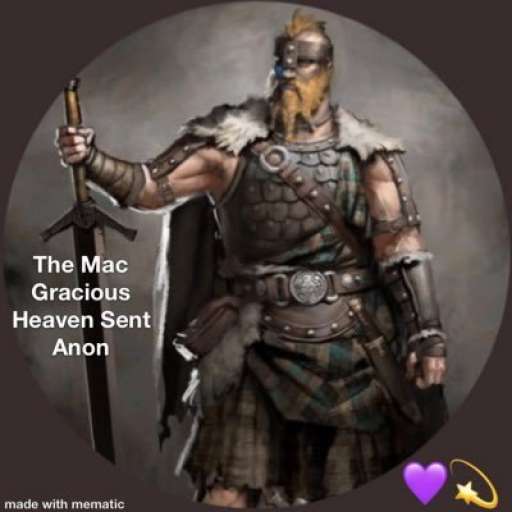
When the ion energy is in the range of a few tens of keV (kilo-electronvolt) these microprobes are usually called FIB (Focused ion beam). An FIB makes a small portion of the material into a plasma; the analysis is done by the same basic techniques as the ones used in mass spectrometry.
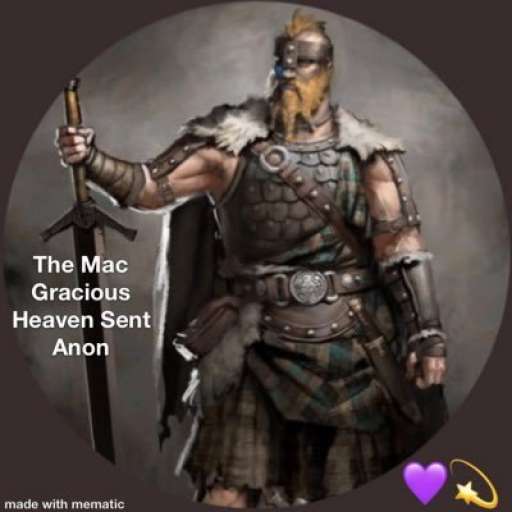
When the ion energy is higher, hundreds of keV to a few MeV (mega-electronvolt) they are called nuclear microprobes. Nuclear microprobes are extremely powerful tools that utilize ion beam analysis techniques as microscopies with spot sizes in the micro-/nanometre range. These instruments are applied to solve scientific problems in a diverse range of fields, from microelectronics to biomedicine. In addition to the development of new ways to exploit these probes as analytical tools (this application area of the nuclear microprobes is called nuclear microscopy), strong progress has been made in the area of materials modification recently (most of which can be described as PBW, proton beam writing)
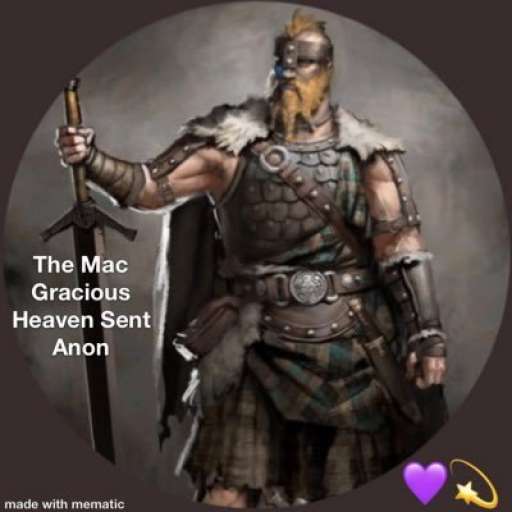
The nuclear microprobe's[5] beam is usually composed of protons and alpha particles. Some of the most advanced nuclear microprobes have beam energies in excess of 2 MeV. This gives the device very high sensitivity to minute concentrations of elements, around 1 ppm at beam sizes smaller than 1 micrometer. This elemental sensitivity exists because when the beam interacts with the a sample it gives off characteristic X-rays of each element present in the sample. This type of detection of radiation is called PIXE. Other analysis techniques are applied to nuclear microscopy including Rutherford backscattering(RBS), STIM, etc.
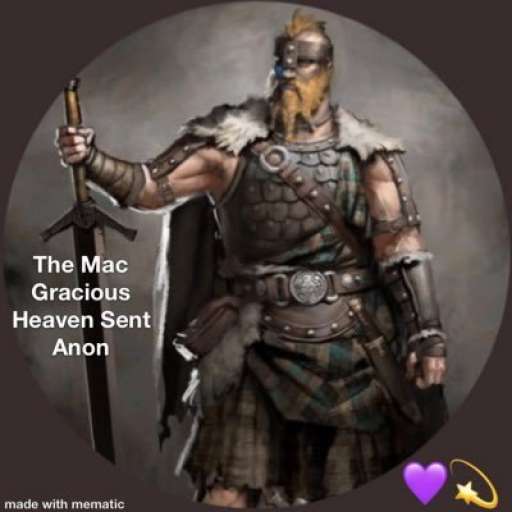
Another use for microprobes is the production of micro and nano sized devices, as in microelectromechanical systems and nanoelectromechanical systems.[6] The advantage that microprobes have over other lithography processes is that a microprobe beam can be scanned or directed over any area of the sample. This scanning of the microprobe beam can be imagined to be like using a very fine tipped pencil to draw your design on a paper or in a drawing program. Traditional lithography processes use photons which cannot be scanned and therefore masks are needed to selectively expose your sample to radiation. It is the radiation that causes changes in the sample, which in turn allows scientists and engineers to develop tiny devices such as microprocessors, accelerometers (like in most car safety systems), etc.
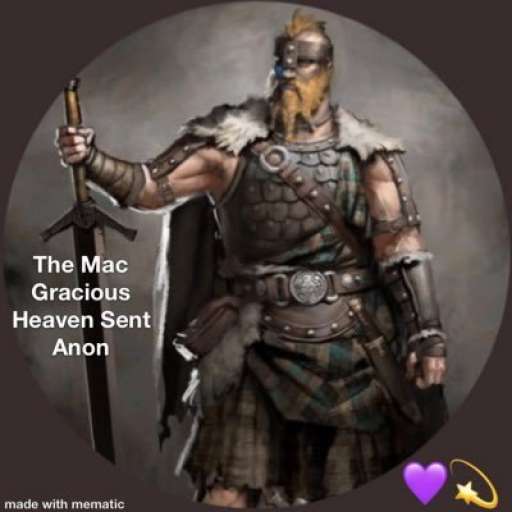
A smart key is an electronic access and authorization system that is available either as standard equipment, or as an option in several car designs. It was first developed by Siemens in 1995 and introduced by Mercedes-Benz under the name "Keyless-Go" in 1998 on the W220 S-Class, after the design patent was filed by Daimler-Benz on May 17, 1997.
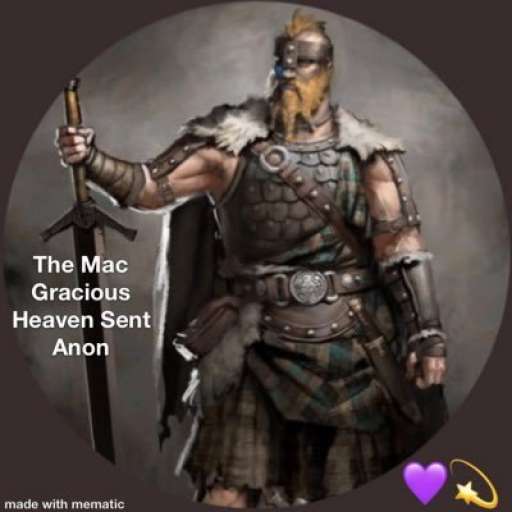
The smart key allows the driver to keep the key fob pocketed when unlocking, locking and starting the vehicle. The key is identified via one of several antennas in the car's bodywork and a radio pulse generator in the key housing. Depending on the system, the vehicle is automatically unlocked when a button or sensor on the door handle or trunk release is pressed. Vehicles with a smart-key system have a mechanical backup, usually in the form of a spare key blade supplied with the vehicle. Some manufacturers hide the backup lock behind a cover for styling.
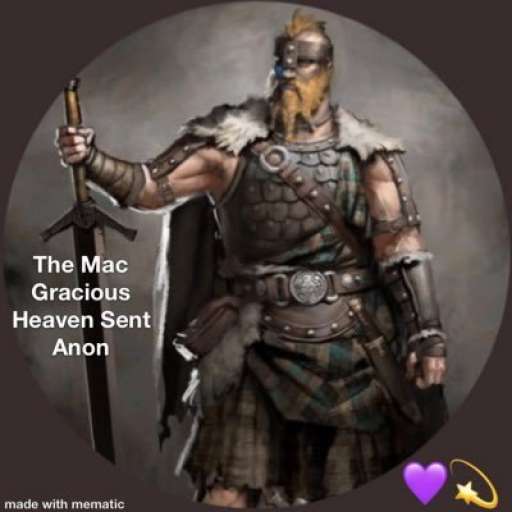
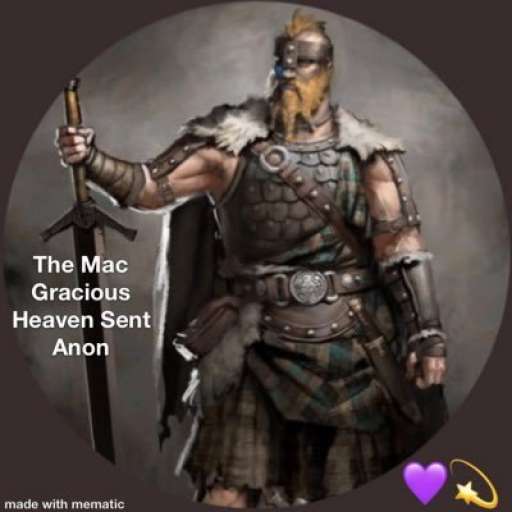
blade; plural noun: blades; noun: blade bone; plural noun: blade bones
1.
the flat cutting edge of a knife, saw, or other tool or weapon.
"cut cleanly using a sharp blade"
LITERARY
a sword.
AUSTRALIAN•NEW ZEALAND
hand shears used in sheep shearing.
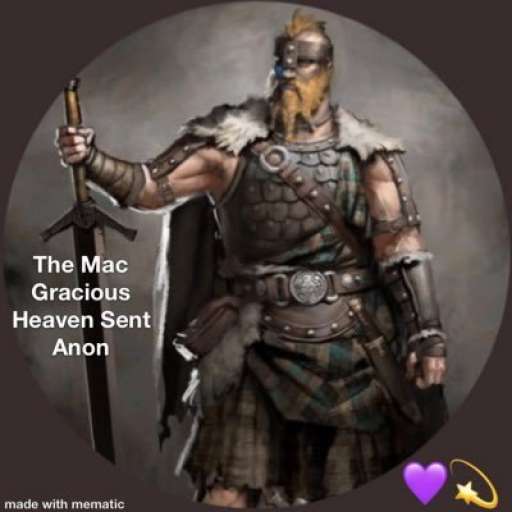
https://en.m.wikipedia.org/wiki/Shear_stress
https://en.m.wikipedia.org/wiki/Shear_force
https://en.m.wikipedia.org/wiki/Compression_(physics)
Technically, a material is under a state of compression, at some specific point and along a specific direction
x
x, if the normal component of the stress vector across a surface with normal direction
x
x is directed opposite to
x
x. If the stress vector itself is opposite to
x
x, the material is said to be under normal compression or pure compressive stress along
x
x. In a solid, the amount of compression generally depends on the direction
x
x, and the material may be under compression along some directions but under traction along others. If the stress vector is purely compressive and has the same magnitude for all directions, the material is said to be under isotropic or hydrostatic compression at that point. This is the only type of static compression that liquids and gases can bear.
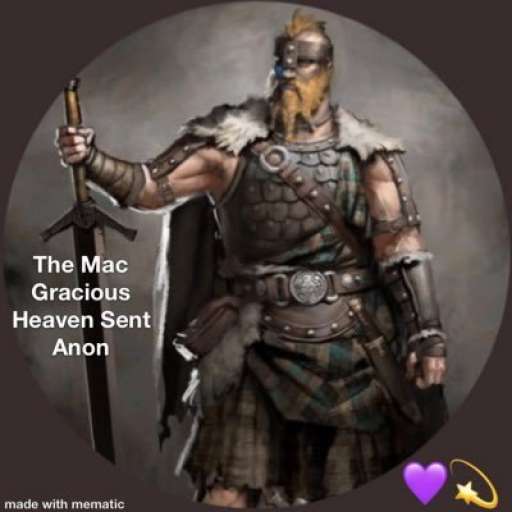
In a mechanical wave which is longitudinal, the medium is displaced in the wave's direction, resulting in areas of compression and rarefaction
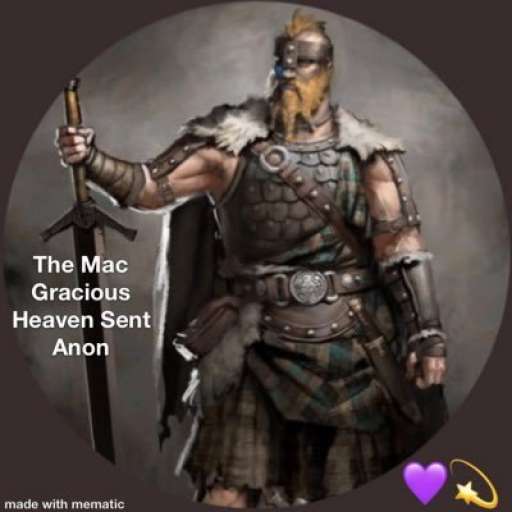
A mechanical wave is a wave that is an oscillation of matter, and therefore transfers energy through a medium.[1] While waves can move over long distances, the movement of the medium of transmission—the material—is limited. Therefore, the oscillating material does not move far from its initial equilibrium position. Mechanical waves transport energy. This energy propagates in the same direction as the wave. Any kind of wave (mechanical or electromagnetic) has a certain energy. Mechanical waves can be produced only in media which possess elasticity and inertia.
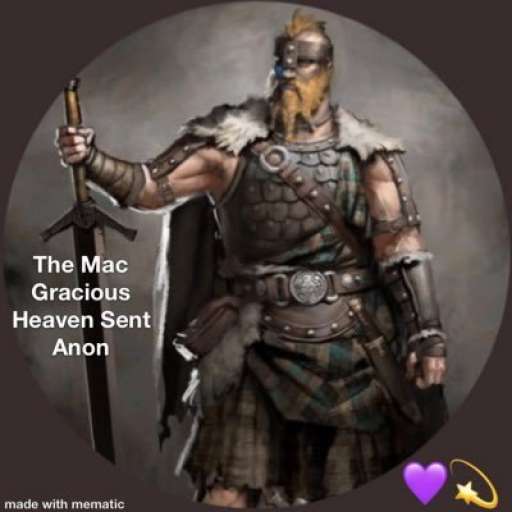
A wave requires an initial energy input. Once this initial energy is added, the wave travels through the medium until all its energy is transferred. In contrast, electromagnetic waves require no medium, but can still travel through one.
One important property of mechanical waves is that their amplitudes are measured in an unusual way, displacement divided by (reduced) wavelength. When this gets comparable to unity, significant nonlinear effects such as harmonic generation may occur, and, if large enough, may result in chaotic effects. For example, waves on the surface of a body of water break when this dimensionless amplitude exceeds 1, resulting in a foam on the surface and turbulent mixing.
There are three types of mechanical waves: transverse waves, longitudinal waves, and surface waves, etc. Some of the most common examples of mechanical waves are water waves, sound waves, and seismic waves.
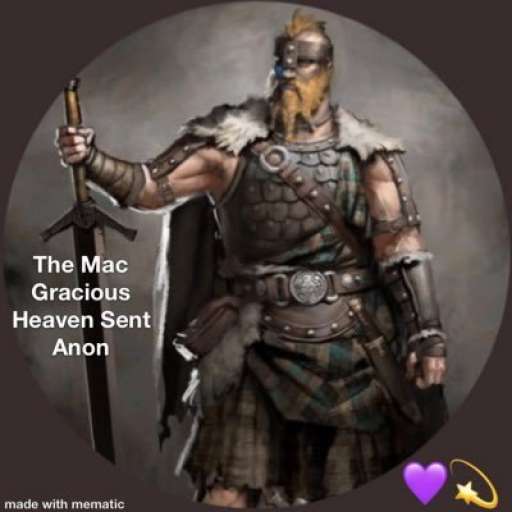
Transverse wave is the form of wave in which particles of medium vibrates about their mean position perpendicular to the direction of the motion of the wave.
To see an example, move an end of a Slinky (whose other end is fixed) to the left-and-right of the Slinky, as opposed to to-and-fro.[2] Light also has properties of a transverse wave, although it is an electromagnetic wave.[3]
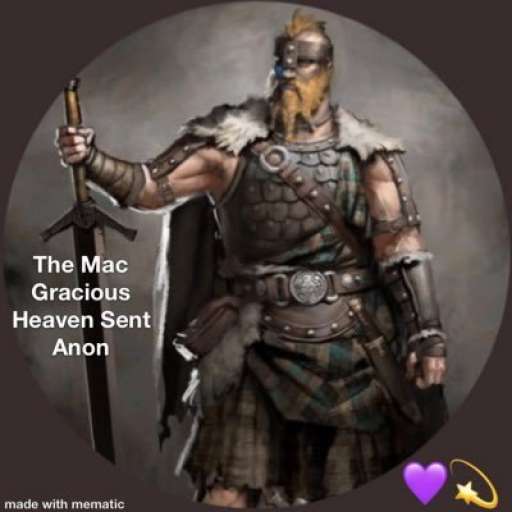
Longitudinal waves cause the medium to vibrate parallel to the direction of the wave. It consists of multiple compressions and rarefactions. The rarefaction is the farthest distance apart in the longitudinal wave and the compression is the closest distance together. The speed of the longitudinal wave is increased in higher index of refraction, due to the closer proximity of the atoms in the medium that is being compressed. Sound is a longitudinal wave.
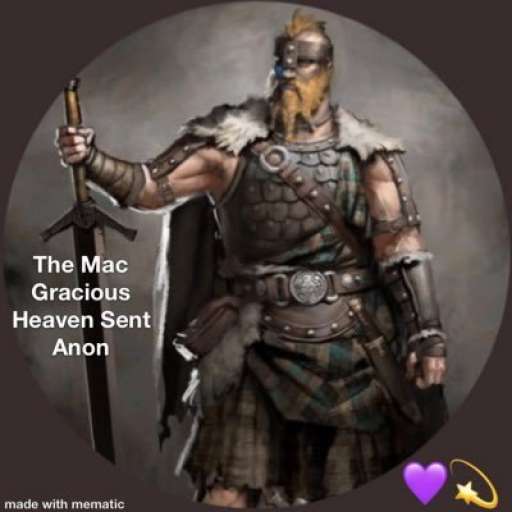
This type of wave travels along the surface or interface between two media. An example of a surface wave would be waves in a pool, or in an ocean, lake, or any other type of water body. There are two types of surface waves, namely Rayleigh waves and Love waves.
Rayleigh waves, also known as ground roll, are waves that travel as ripples with motion similar to those of waves on the surface of water. Such waves are much slower than body waves, at roughly 90% of the velocity of bulk waves[clarify] for a typical homogeneous elastic medium. Rayleigh waves have energy losses only in two dimensions and are hence more destructive in earthquakes than conventional bulk waves, such as P-waves and S-waves, which lose energy in all three directions.
A Love wave is a surface wave having horizontal waves that are shear or transverse to the direction of propagation. They usually travel slightly faster than Rayleigh waves, at about 90% of the body wave velocity, and have the largest amplitude.
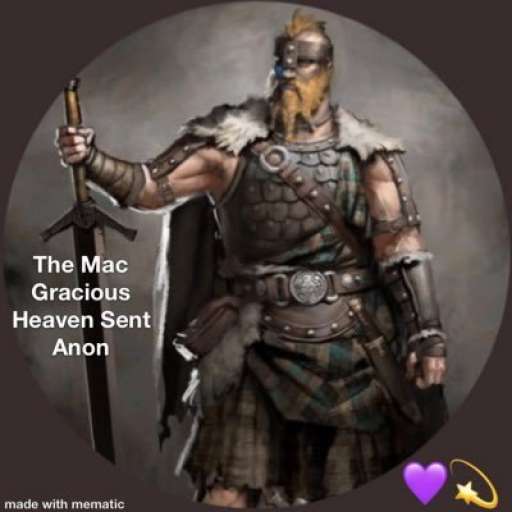
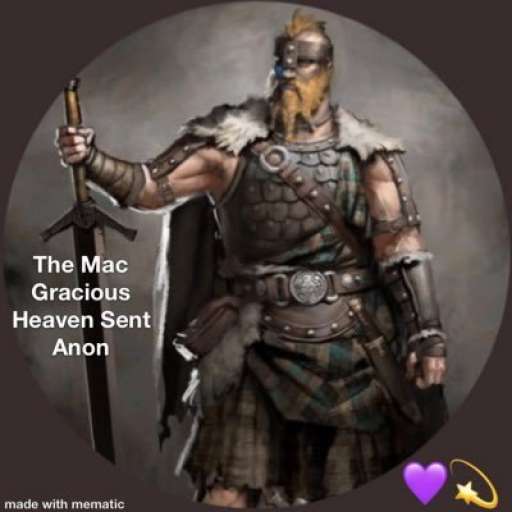
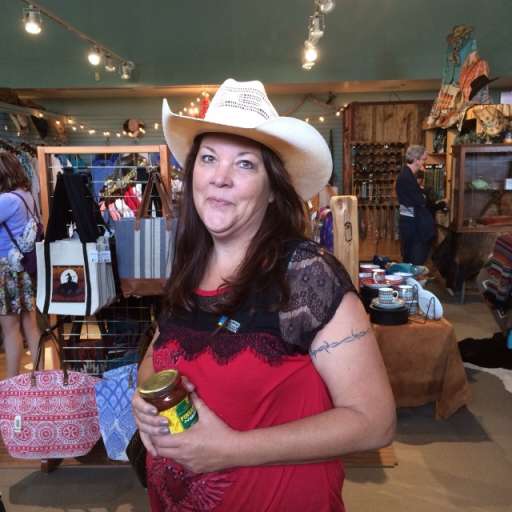
so could sound or music at the same frequency?
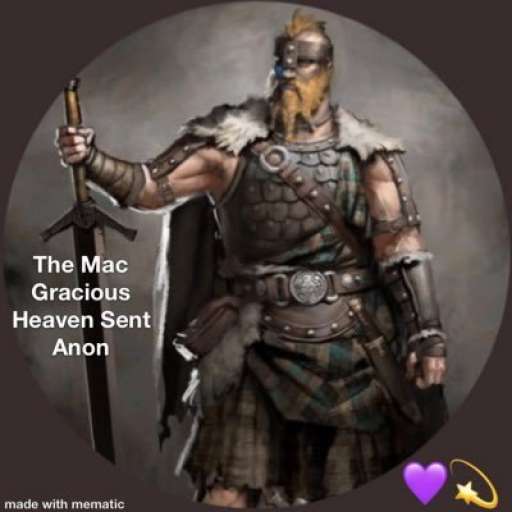
You have hit the right note.
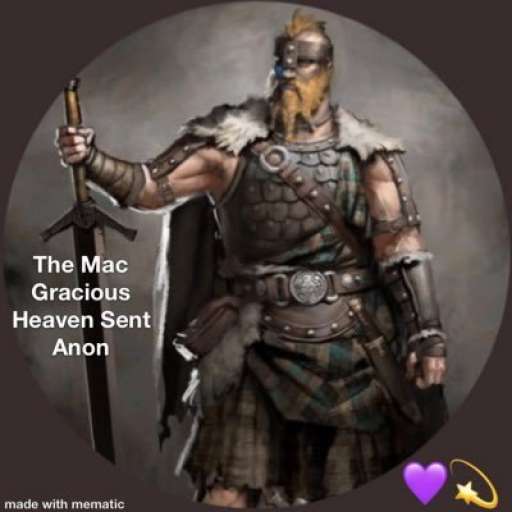
un·der·tone
(ŭn′dər-tōn′) 1. An underlying or implied tendency or meaning; an undercurrent. 2. A tone of low pitch or volume, especially of spoken sound.
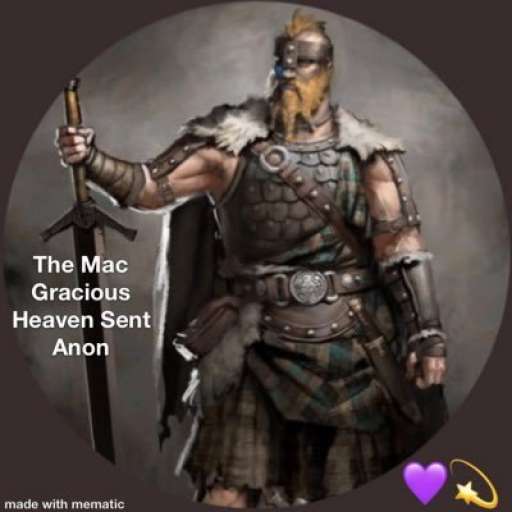
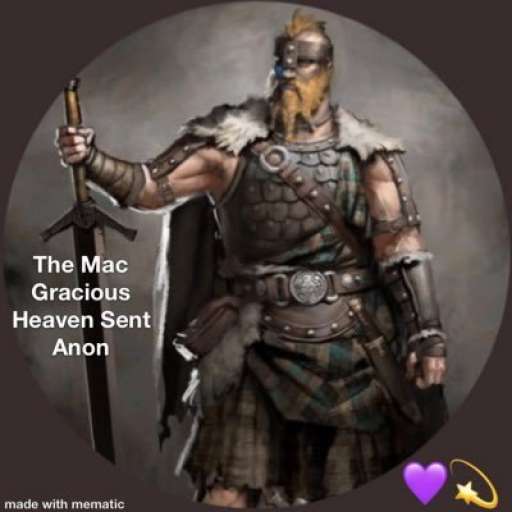
Tonic water (or Indian tonic water) is a carbonated soft drink in which quinine is dissolved. Originally used as a prophylactic against malaria, tonic water usually now has a significantly lower quinine content and is consumed for its distinctive bitter flavor, though it is nowadays also often sweetened. It is often used in mixed drinks, particularly in gin and tonic.
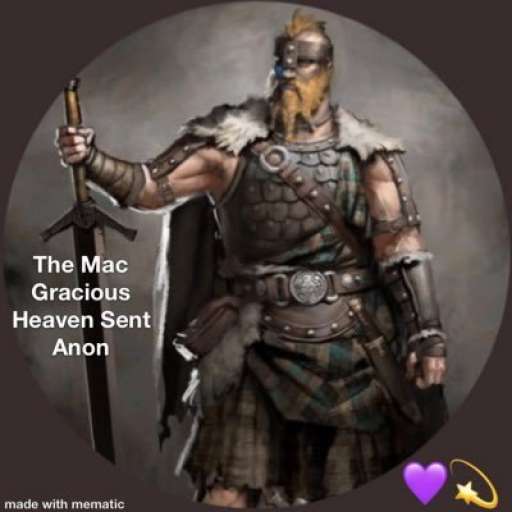
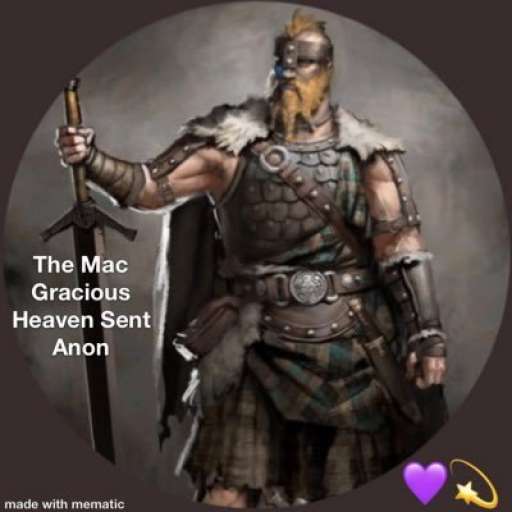
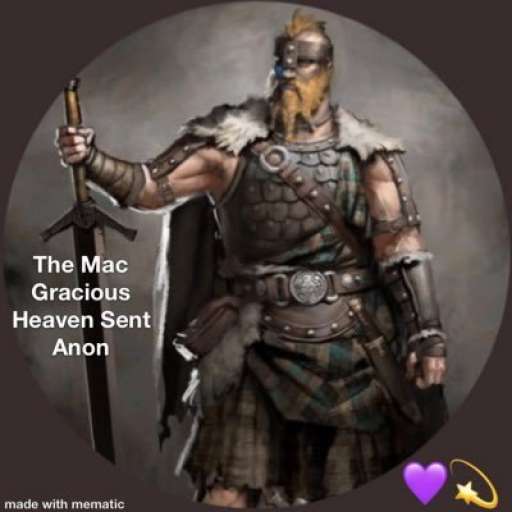
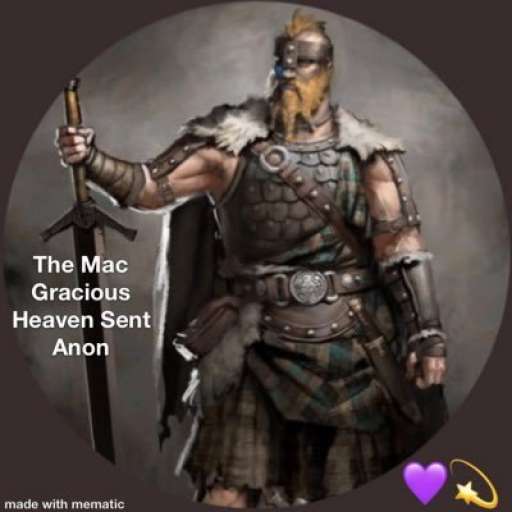
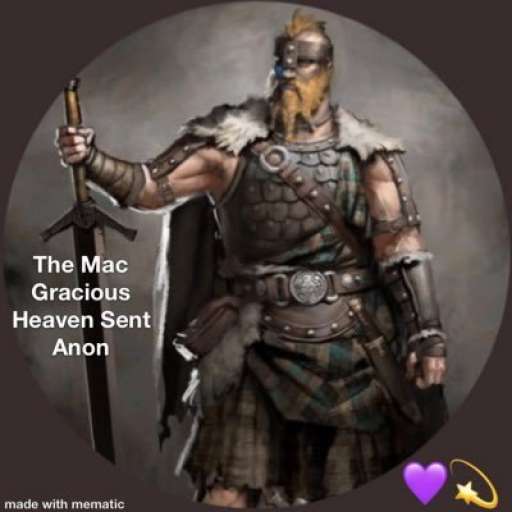
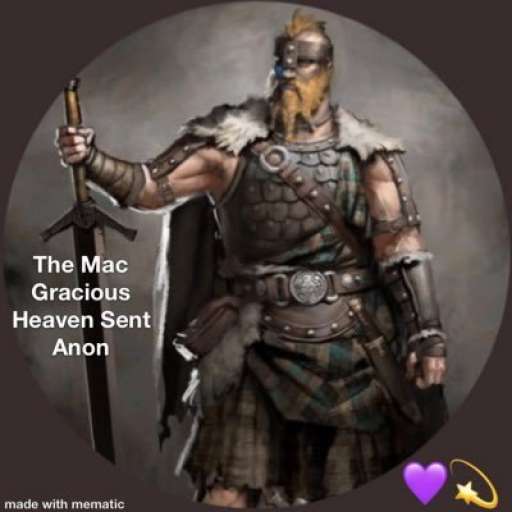
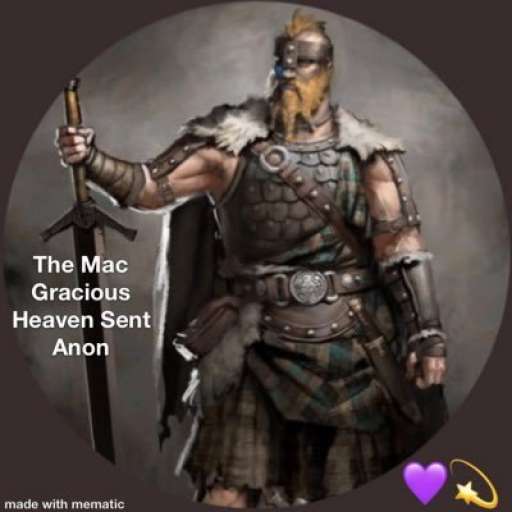
The ordering of synthetic liquid crystals near surfaces is known to be dependent on the nanoscopic structure and chemical functionality of surfaces. In this letter, we report that the orientational ordering of synthetic liquid crystals on surfaces decorated with viruses is also dependent on the structures of the viruses. Each of the four virions investigated had diameters of approximately 100 nm, but three of the viruses (influenza virus, La Crosse virus, and vesicular stomatitis virus) were enveloped in a lipid bilayer, whereas one virus (adenovirus) was not.
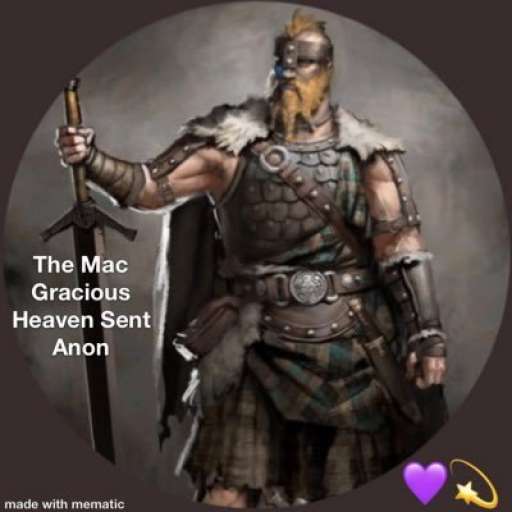
We observed that lipid bilayer-enveloped viruses induce homeotropic (perpendicular) ordering of a nematic liquid crystal upon contact with the liquid crystal. In contrast, nonenveloped virus (adenovirus)-treated surfaces caused a near-planar orientation of the liquid crystal. We conclude that the homeotropic ordering of liquid crystals is a signature of the presence of enveloped viruses present on surfaces. These results suggest new approaches to the design of nanostructured materials that incorporate viruses as well as suggest methods that can be used to amplify the presence of nanoscopic virions into micrometer-sized domains of liquid crystal that can be optically probed.
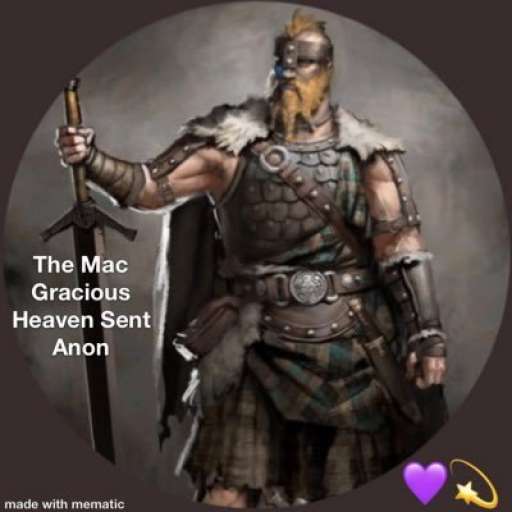
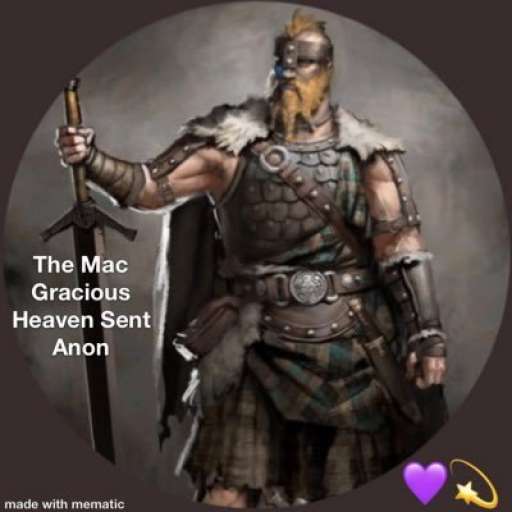
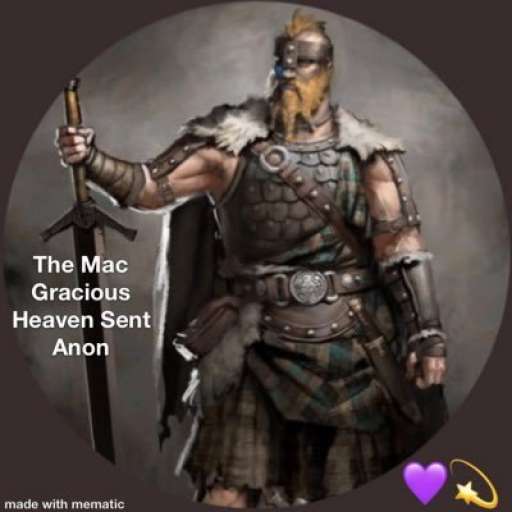
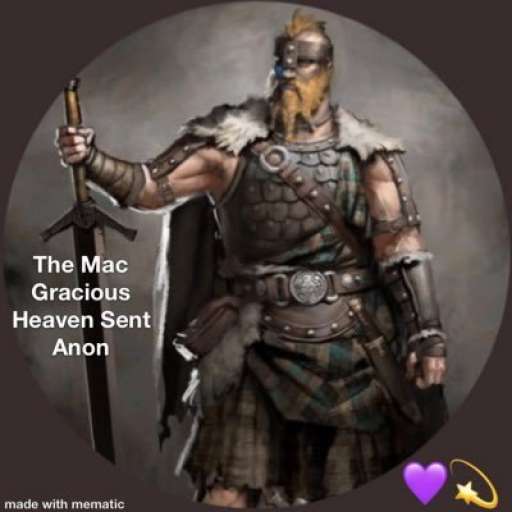
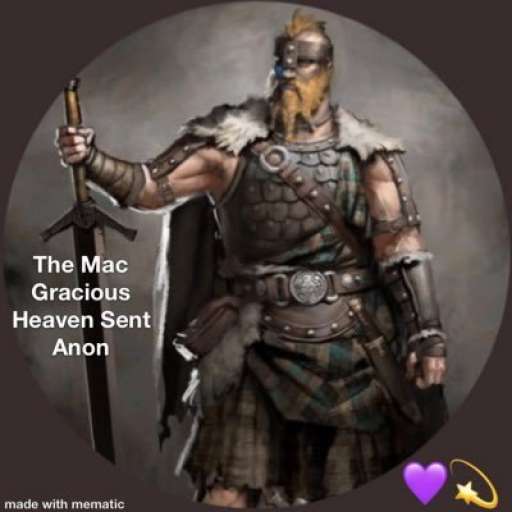
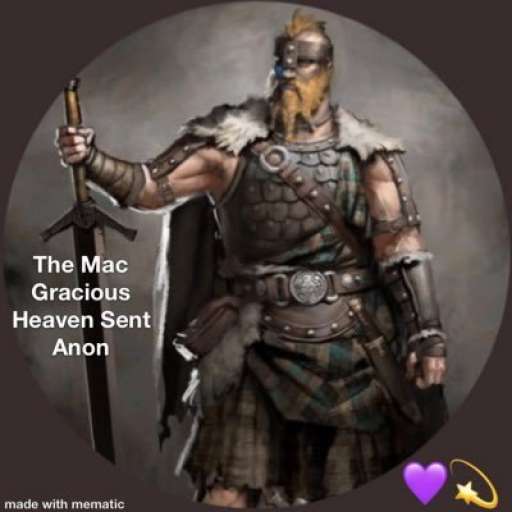
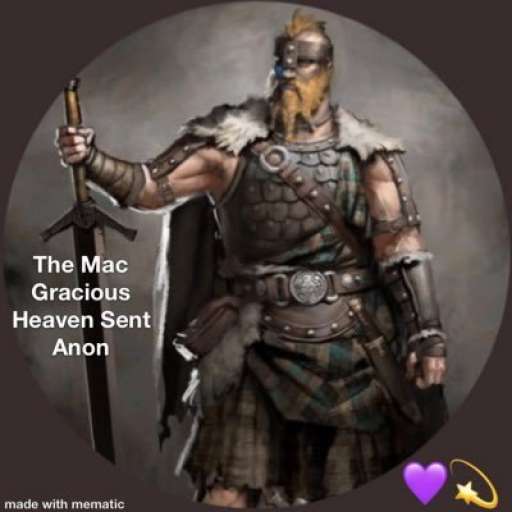
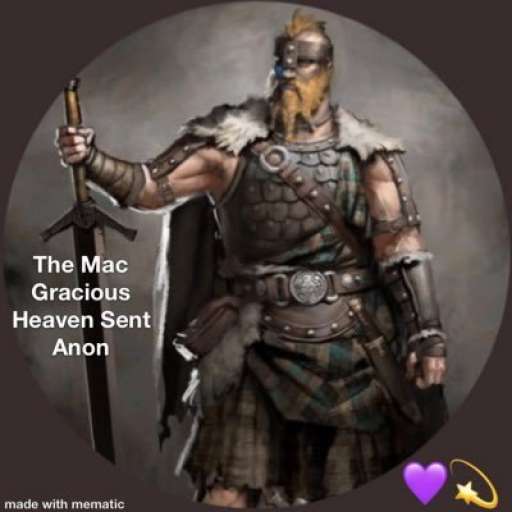
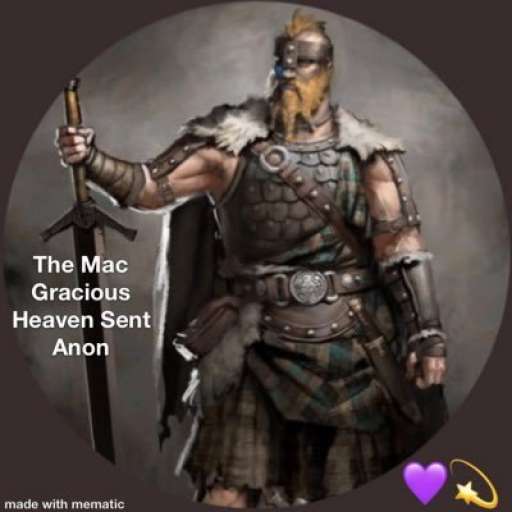
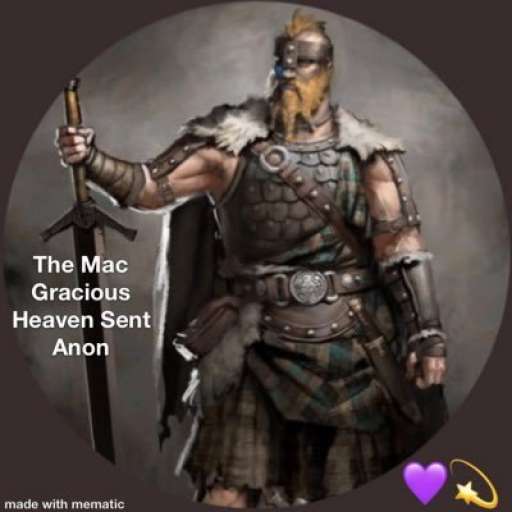
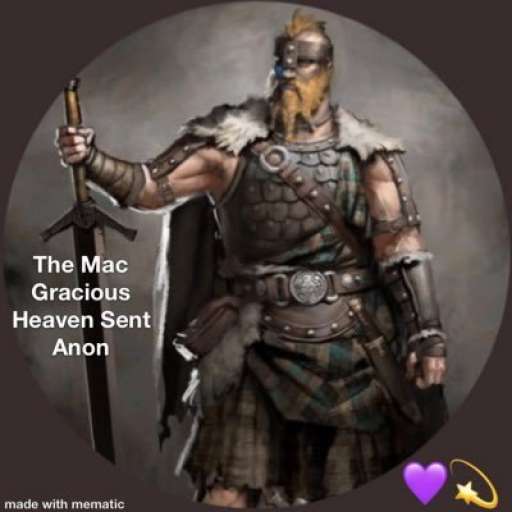
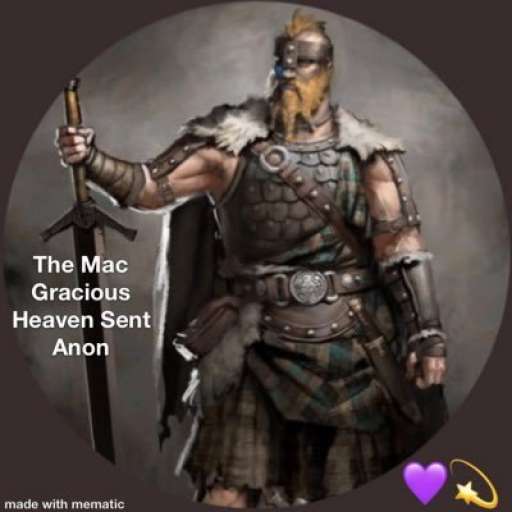
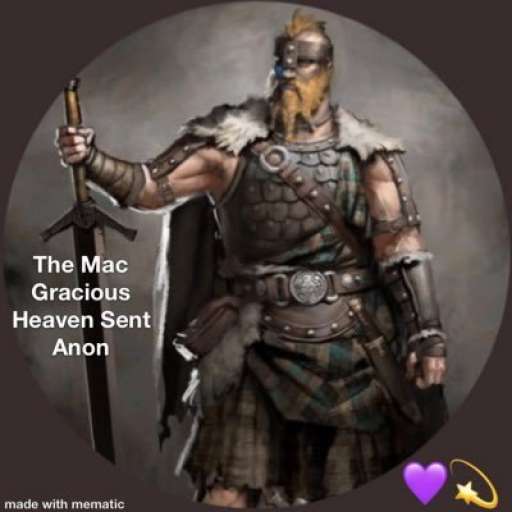
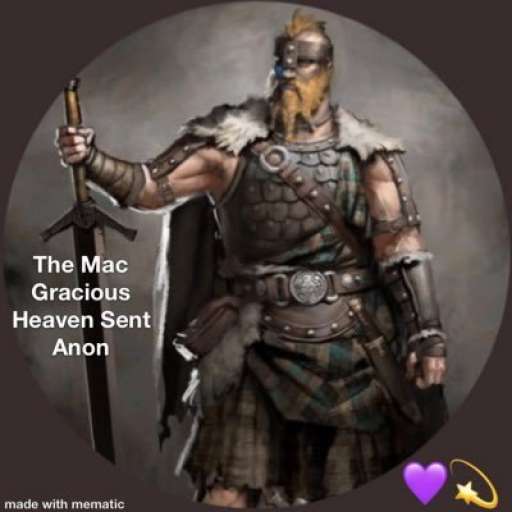
A microlens is a small lens, generally with a diameter less than a millimetre (mm) and often as small as 10 micrometres (µm).
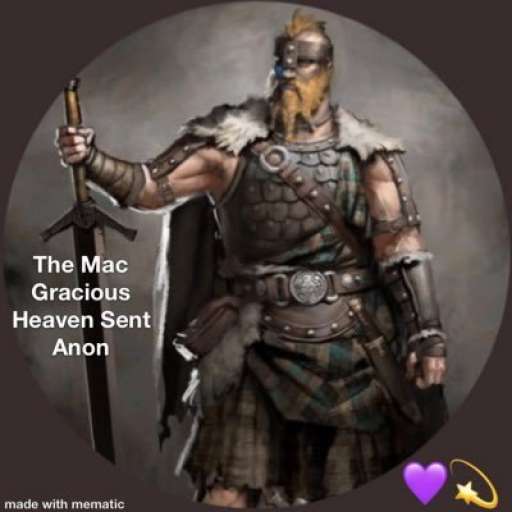
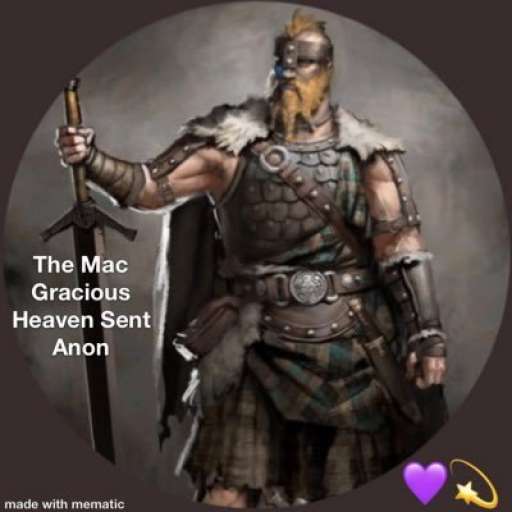
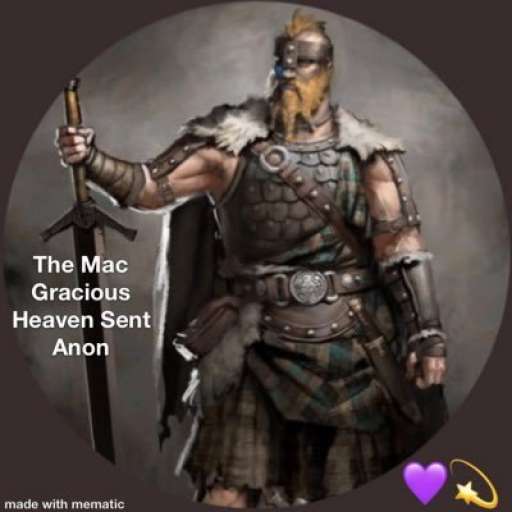
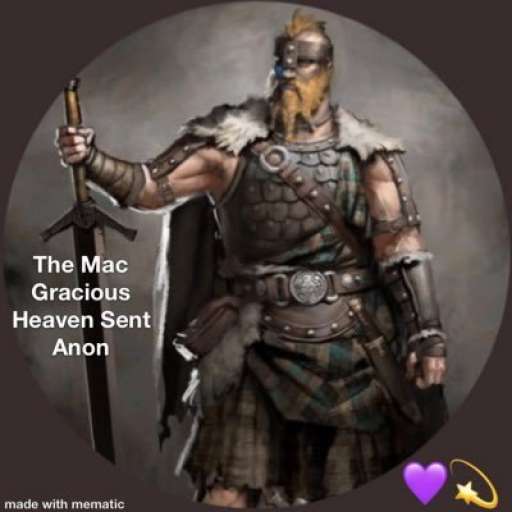
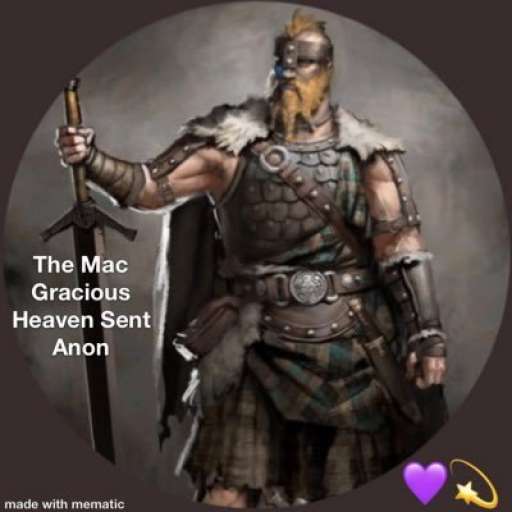
Arrays of metal nanoparticles in an organic matrix have attracted a lot of interest due to their diverse electronic and optoelectronic properties. By varying parameters such as the nanoparticle material, the matrix material, the nanoparticle size, and the interparticle distance, the electronic behavior of the nanoparticle array can be substantially tuned and controlled. For strong tunnel coupling between adjacent nanoparticles, the assembly exhibits conductance properties similar to the bulk properties of the nanoparticle material. When the coupling between the nanoparticles is reduced, a metal insulator transition is observed in the overall assembly. Recent work demonstrates that nanoparticle arrays can be further utilized to incorporate single molecules, such that the nanoparticles act as electronic contacts to the molecules. Furthermore, via the excitation of the surface plasmon polaritons, the nanoparticles can be optically excited and electronically read out.
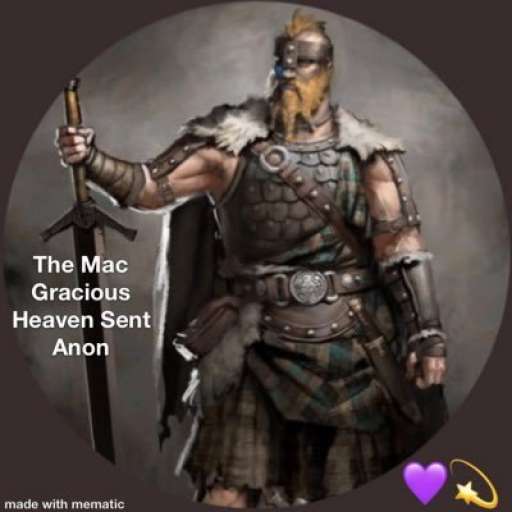
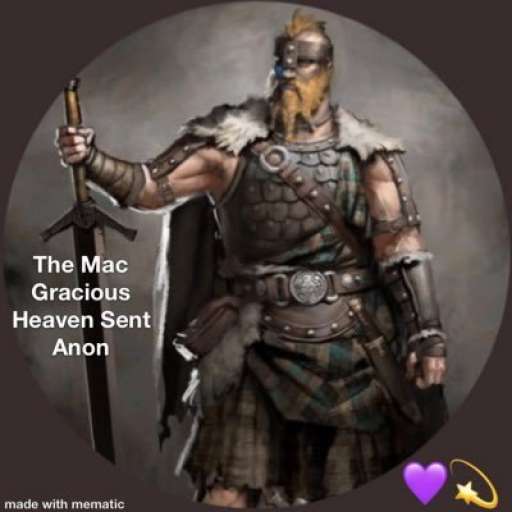
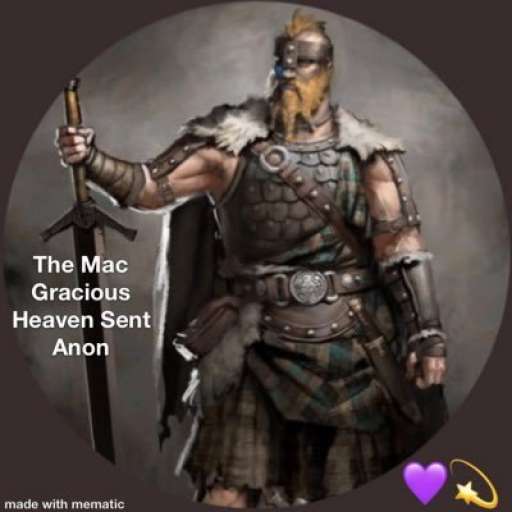
Homogeneous nucleation is responsible for the formation of particles from the vapor phase. In this process, vapor atoms or molecules are transformed into solid particles in a supersaturated vapor without the presence of a foreign phase.
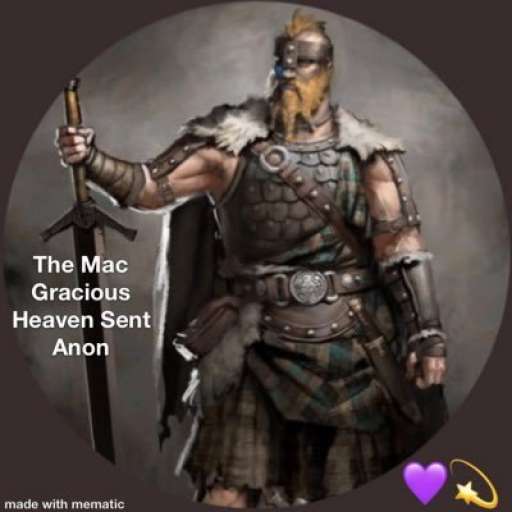
Homogeneous and heterogeneous nucleation are the two major forms of nucleation. The difference between homogeneous and heterogeneous nucleation is that homogeneous nucleation occurs away from the surface of the system whereas heterogeneous nucleation occurs at the surface of the system
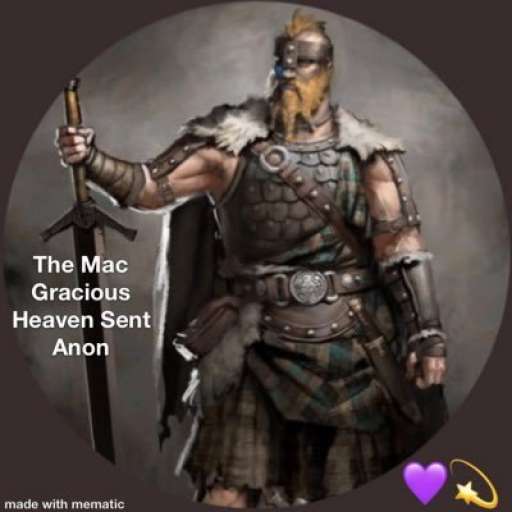
(of a plastic solid) undergo gradual deformation under stress.
In materials science, creep (sometimes called cold flow) is the tendency of a solid material to move slowly or deform permanently under the influence of persistent mechanical stresses. It can occur as a result of long-term exposure to high levels of stress that are still below the yield strength of the material. Creep is more severe in materials that are subjected to heat for long periods and generally increases as they near their melting point.
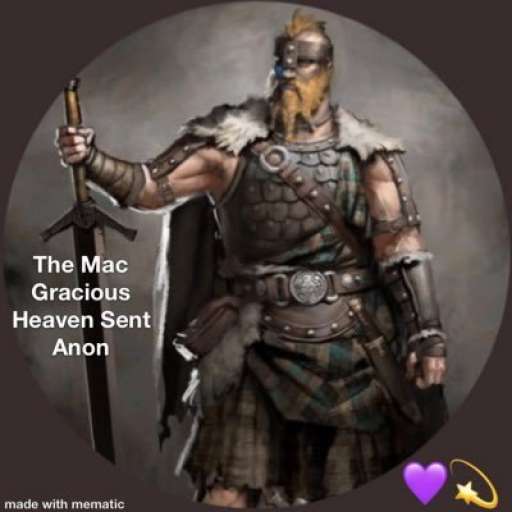
Creep behavior can be split into three main stages. In primary, or transient, creep, the strain rate is a function of time. In Class M materials, which include most pure materials, strain rate decreases over time. This can be due to increasing dislocation density, or it can be due to evolving grain size. In class A materials, which have large amounts of solid solution hardening, strain rate increases over time due to a thinning of solute drag atoms as dislocations move.
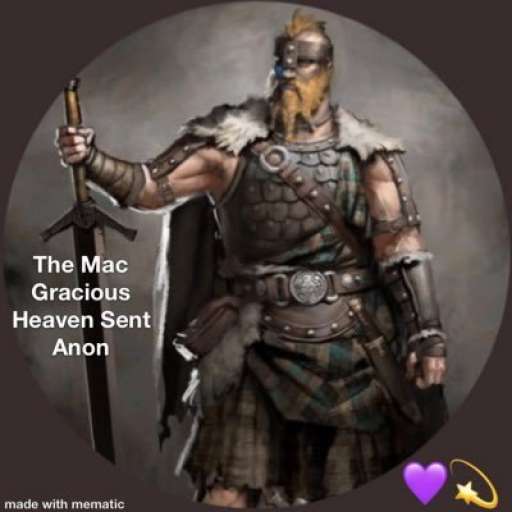
In the secondary, or steady-state, creep, dislocation structure and grain size have reached equilibrium, and therefore strain rate is constant. Equations that yield a strain rate refer to the steady-state strain rate. Stress dependence of this rate depends on the creep mechanism.
In tertiary creep, the strain rate exponentially increases with stress. This can be due to necking phenomena, internal cracks, or voids, which all decrease the cross-sectional area and increase the true stress on the region, further accelerating deformation and leading to fracture
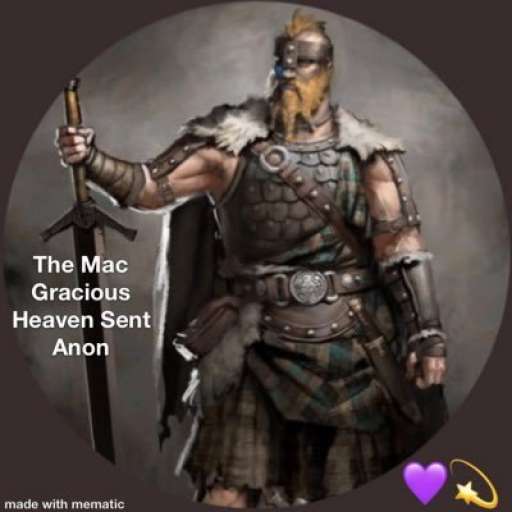
A grain boundary is the interface between two grains, or crystallites, in a polycrystalline material. Grain boundaries are 2D defects in the crystal structure, and tend to decrease the electrical and thermal conductivity of the material. Most grain boundaries are preferred sites for the onset of corrosion[1] and for the precipitation of new phases from the solid. They are also important to many of the mechanisms of creep.[2] On the other hand, grain boundaries disrupt the motion of dislocations through a material, so reducing crystallite size is a common way to improve mechanical strength, as described by the Hall–Petch relationship. The study of grain boundaries and their effects on the mechanical, electrical and other properties of materials forms an important topic in materials science.
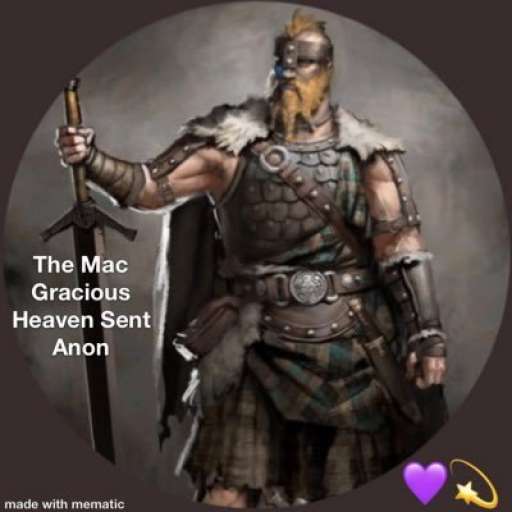
A CRYSTALLITE
🕯
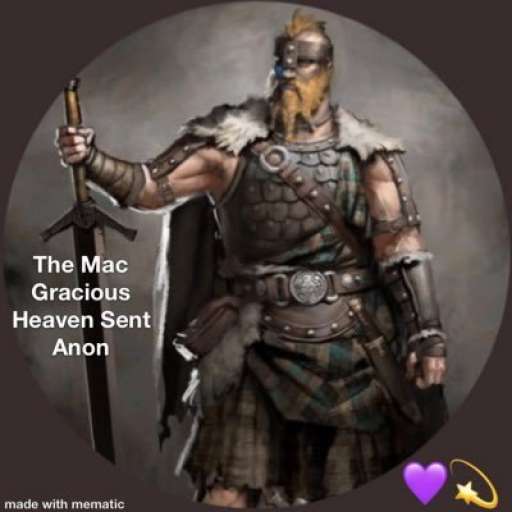
crystallite (plural crystallites)
A small region of a solid that consists of a single crystal; a grain.
Derived terms
intercrystallite
microcrystallite
nanocrystallite
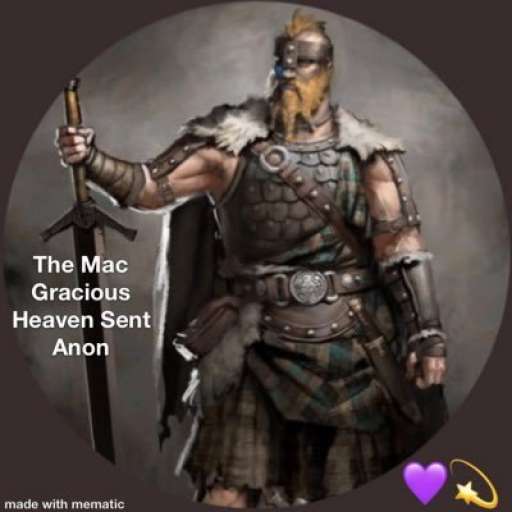
cristallite m (plural cristalliti)
crystallite
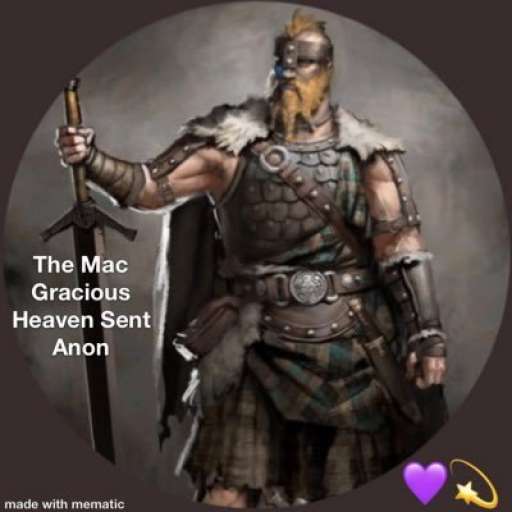
AN ALITTEESEEN
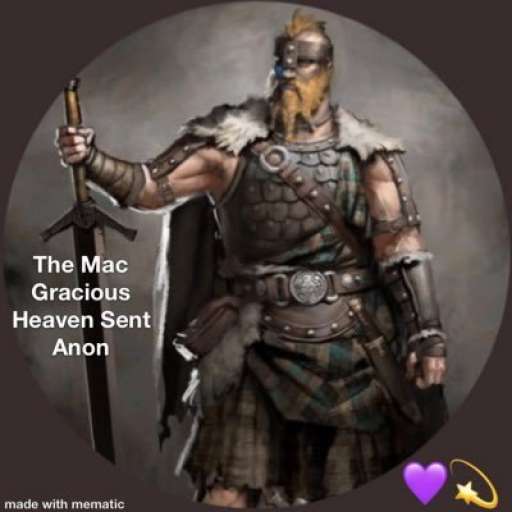
Halite (/ˈhælˌaɪt, ˈheɪˌlaɪt/),[7][8][9][10] commonly known as rock salt, is a type of salt, the mineral (natural) form of sodium chloride (NaCl). Halite forms isometric crystals.
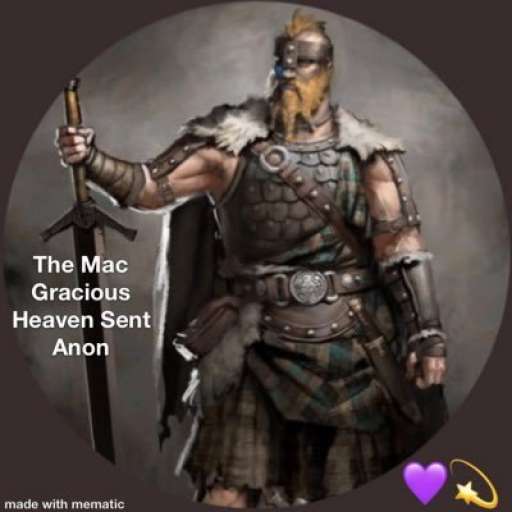
CN103708539B - Preparation method of halite LiTiO2 nanoparticles - Google Patents
The invention relates to a preparation method of halite LiTiO2 nanoparticles. By using K2Ti6O13 nano fiber as a titanium source precursor, lithium nitrate as a lithium source and potassium hydroxide as a mineralizer, a simple one-step hydrothermal reaction process is utilized to prepare the ha..
https://patents.google.com/patent/CN103708539B/en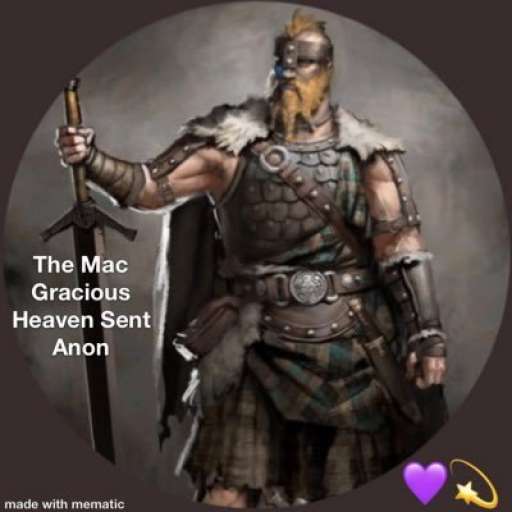
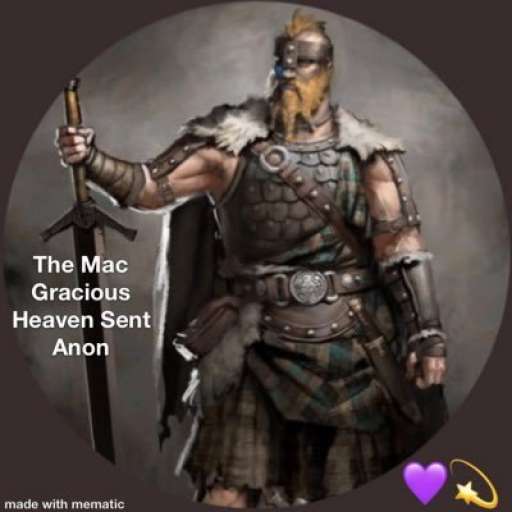
Simple size control of TiO2 nanoparticles and their electrochemical performance: emphasizing the contribution of the surface area to lithium sto..
The particle size effects of TiO2 nanoparticles (TNPs), which are composed of small crystallites, on Li ion storage are a very fundamental and important subject. However, size control of TNPs under 200 nm using a sol–gel method has been limited due to the highly reactive precursor, titanium alkoxide..
https://pubs.rsc.org/en/content/articlelanding/2016/nr/c6nr00104a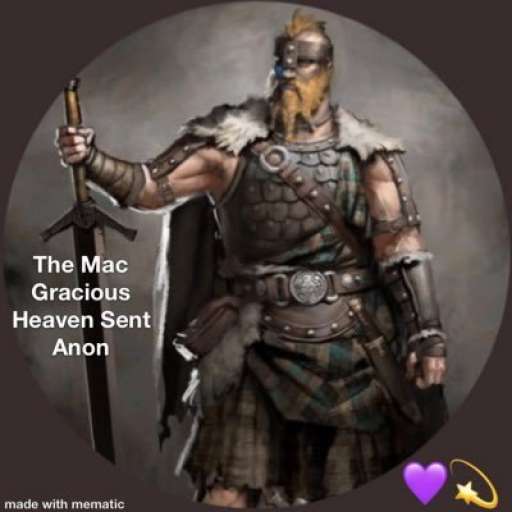
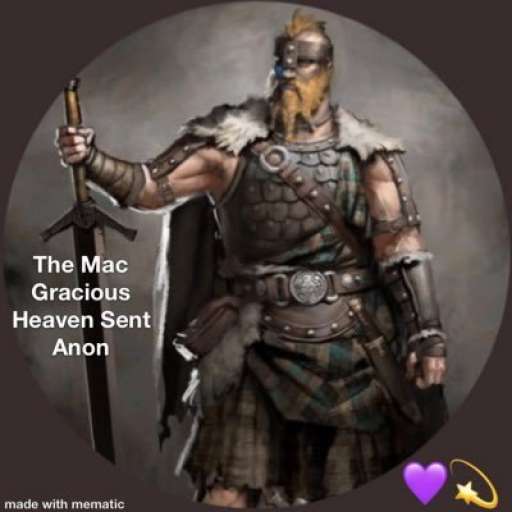
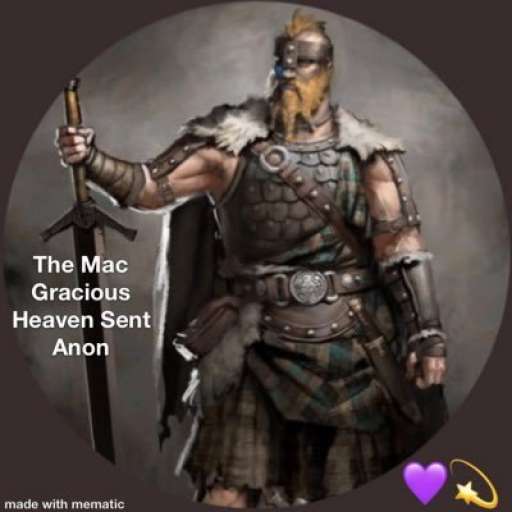
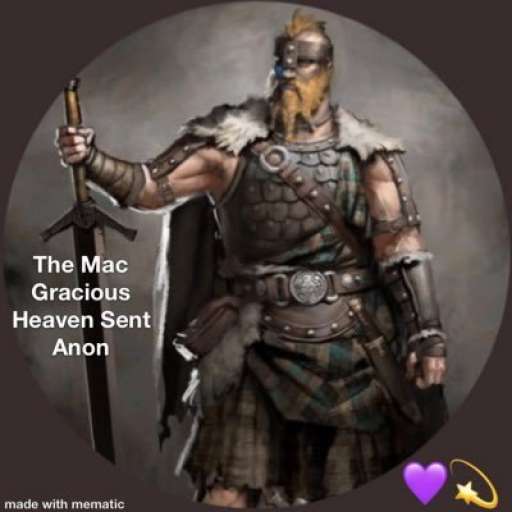
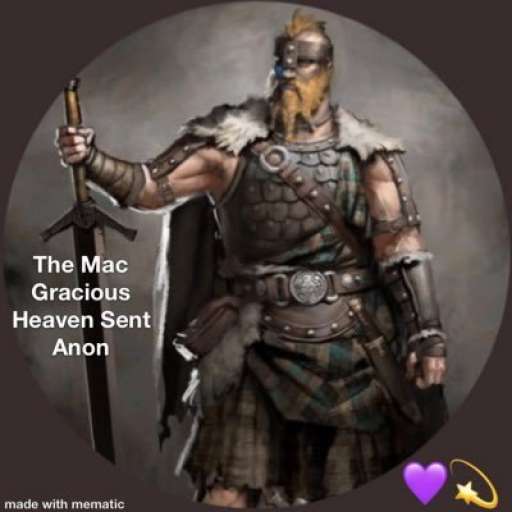
electron volt, unit of energy commonly used in atomic and nuclear physics, equal to the energy gained by an electron (a charged particle carrying unit electronic charge) when the electrical potential at the electron increases by one volt. The electron volt equals 1.602 × 10−12 erg, or 1.602 × 10−19 joule. The abbreviation MeV indicates 106 (1,000,000) electron volts; GeV, 109 (1,000,000,000); and TeV, 1012 (1,000,000,000,000).
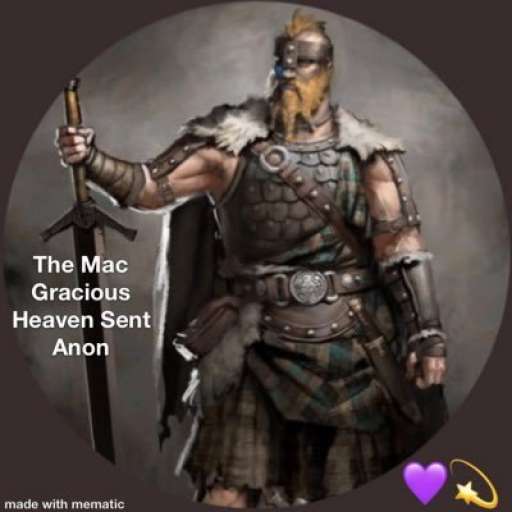
An electronvolt is the amount of kinetic energy gained or lost by a single electron accelerating from rest through an electric potential difference of one volt in vacuum.
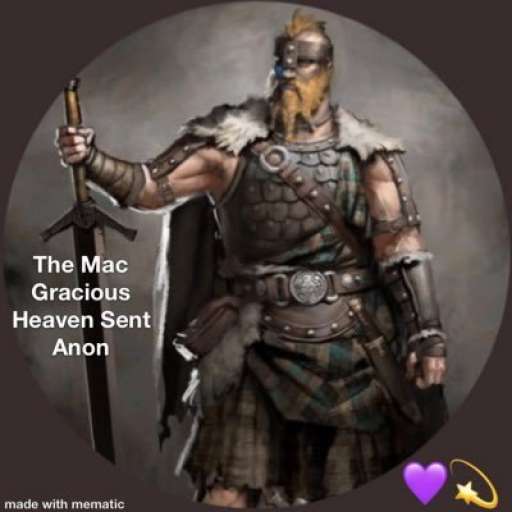
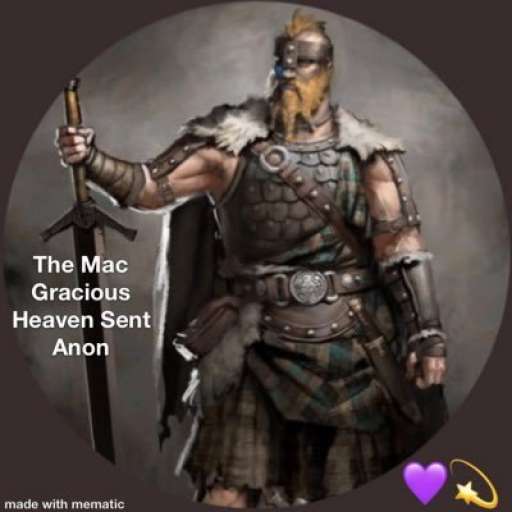
The initial jolt of electricity to the body can affect the central nervous system, motor neurons, and other nerves, as well as their control centers in the brain. These areas are damaged, often leading to a permanent impairment after high voltage exposure.
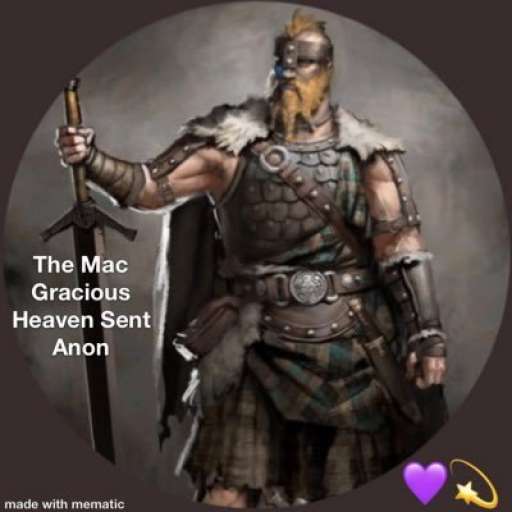
Summary: Researchers have shown that an electric shock ranging from 120 to 52,000 volts can cause neurologic and neuropsychological symptoms in humans. Following an electrical injury, some patients may show various emotional and behavioral aftereffects, such as memory loss and symptoms of depression.
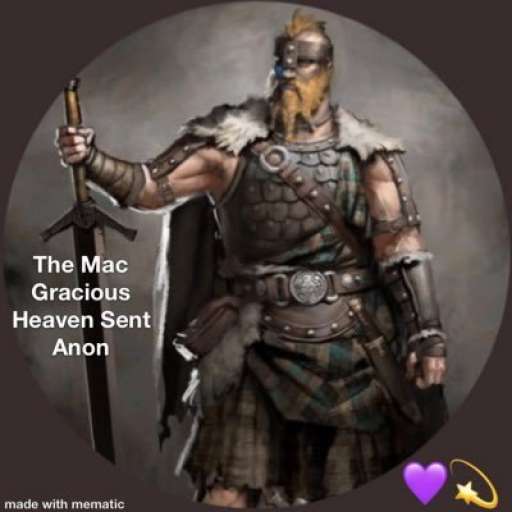
The people are unimpressed with the government.