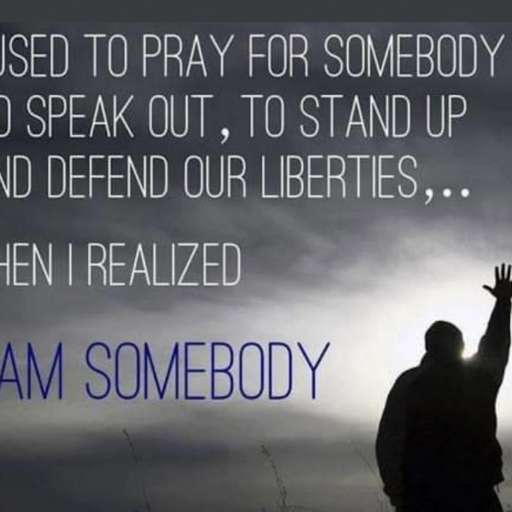
100% Texan Patriot. Lifelong seeker of truth. Love of God, family and the goodness of humanity, let it shine! 🌞
what is this?
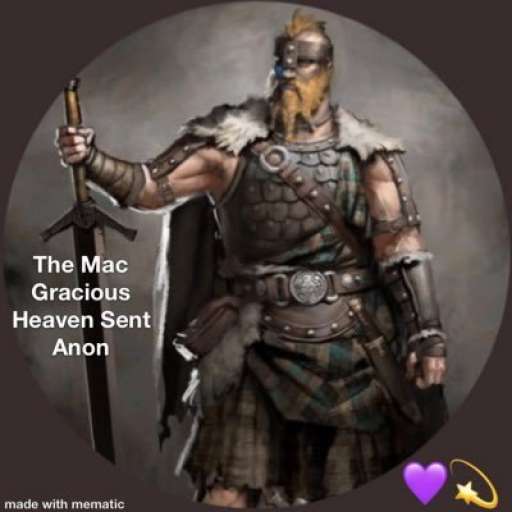
Luminous intensity, the quantity of visible light that is emitted in unit time per unit solid angle. The unit for the quantity of light flowing from a source in any one second (the luminous power, or luminous flux) is called the lumen. The lumen is evaluated with reference to visual sensation.
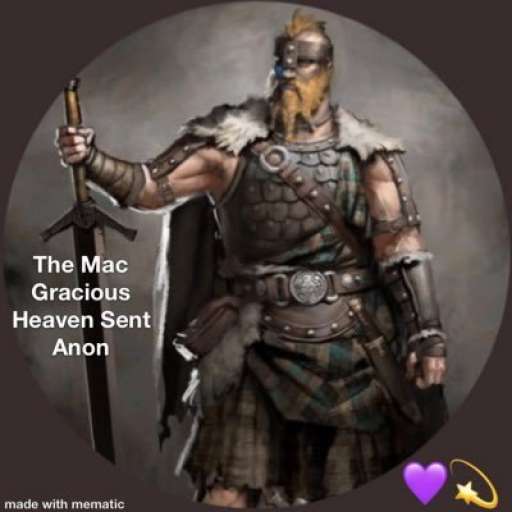
Several measures of light are commonly known as intensity:
Radiant intensity, a radiometric quantity measured in watts per steradian (W/sr)
Luminous intensity, a photometric quantity measured in lumens per steradian (lm/sr), or candela (cd)
Irradiance, a radiometric quantity, measured in watts per square meter (W/m2)
Intensity (physics), the name for irradiance used in other branches of physics (W/m2)
Radiance, commonly called "intensity" in astronomy and astrophysics (W·sr−1·m−2)
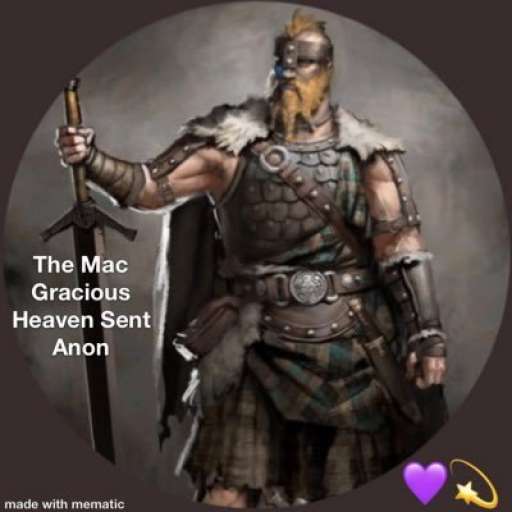
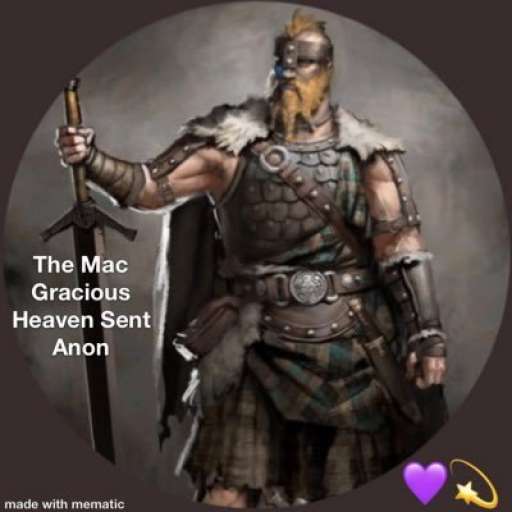
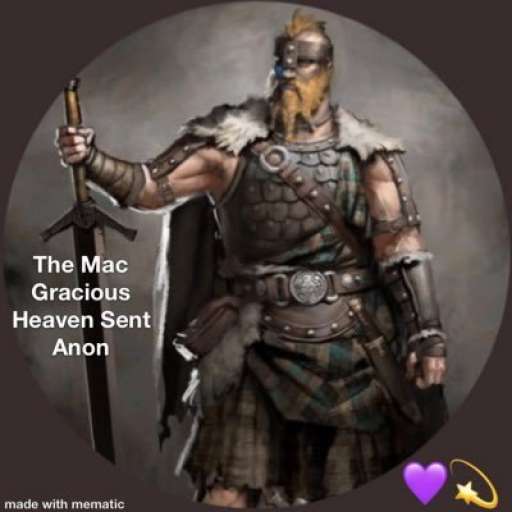
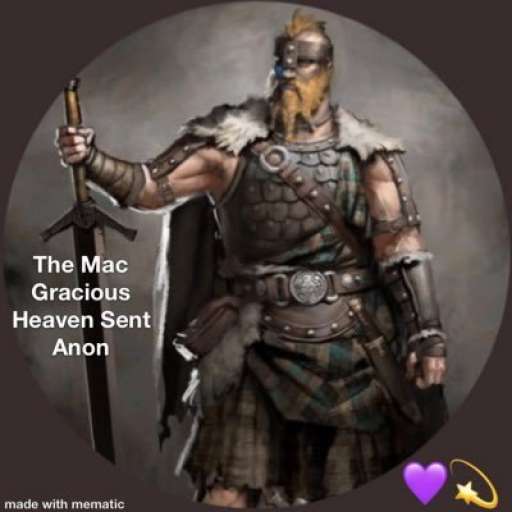
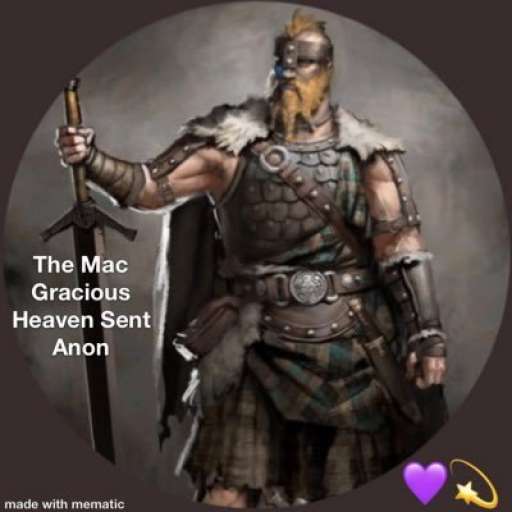
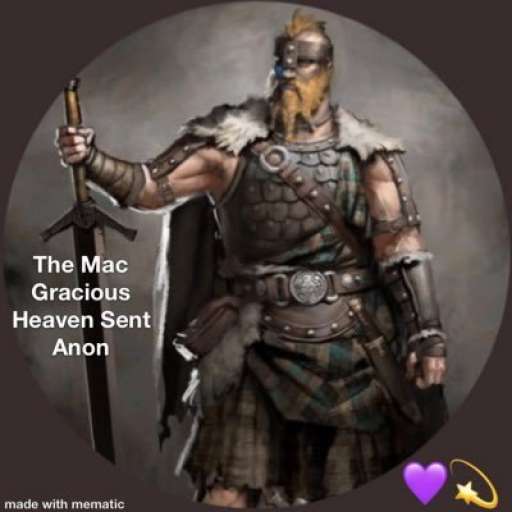
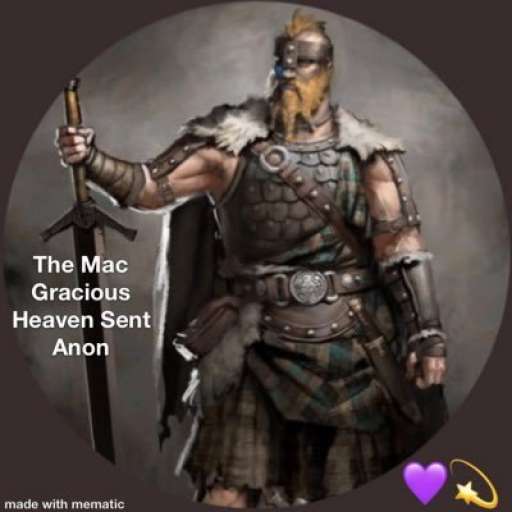
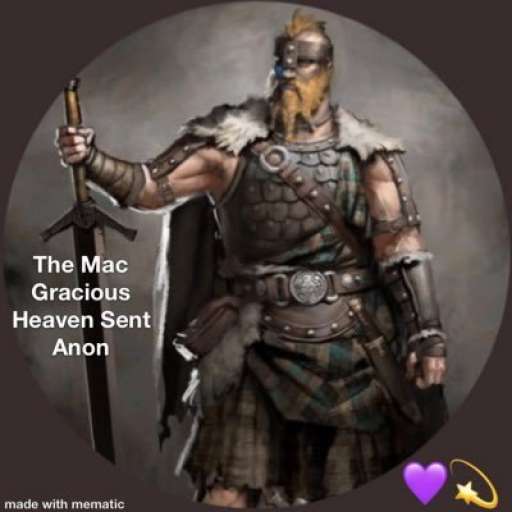
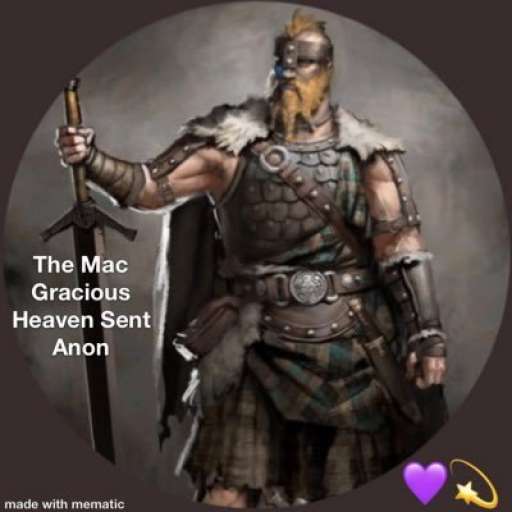
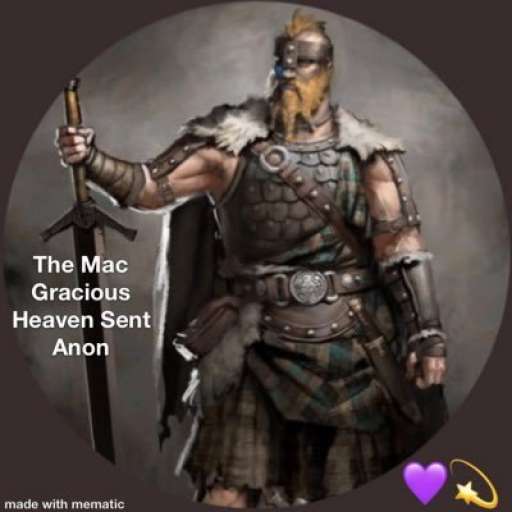
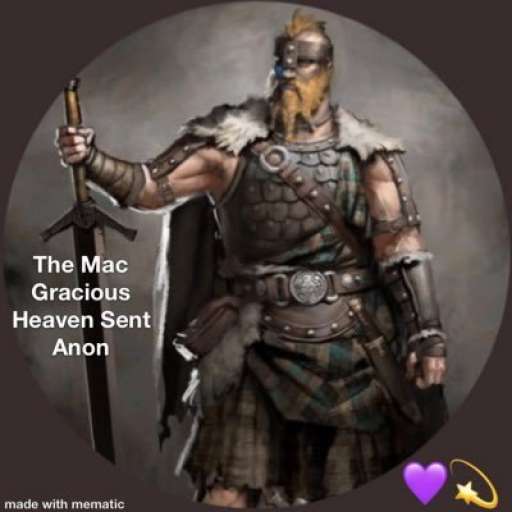
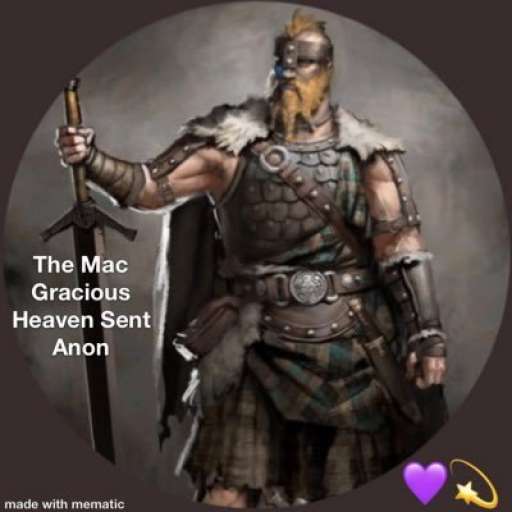
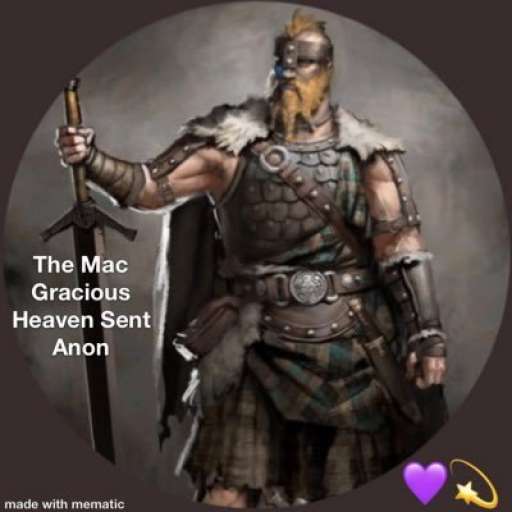
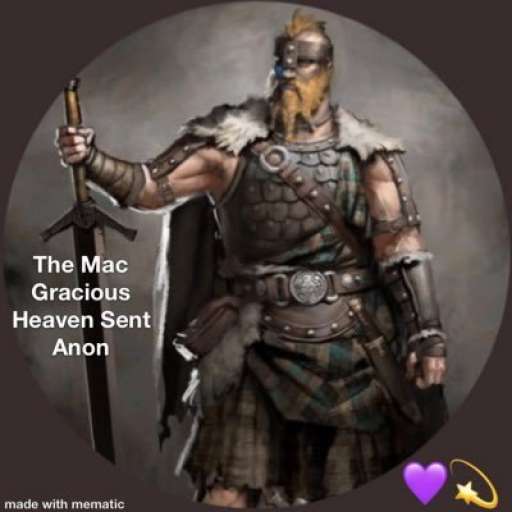
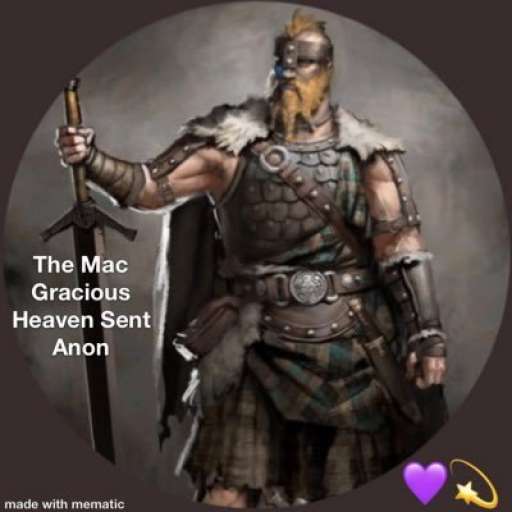
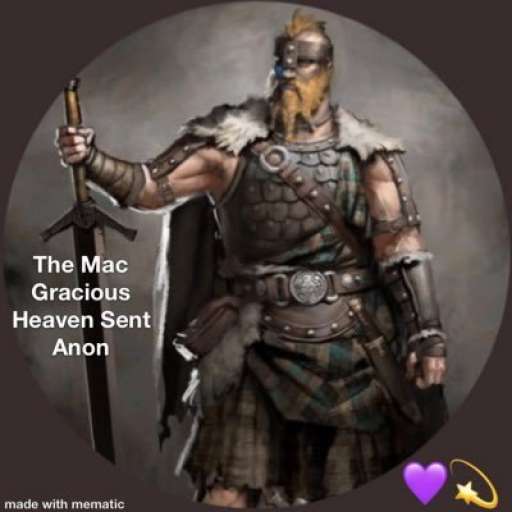
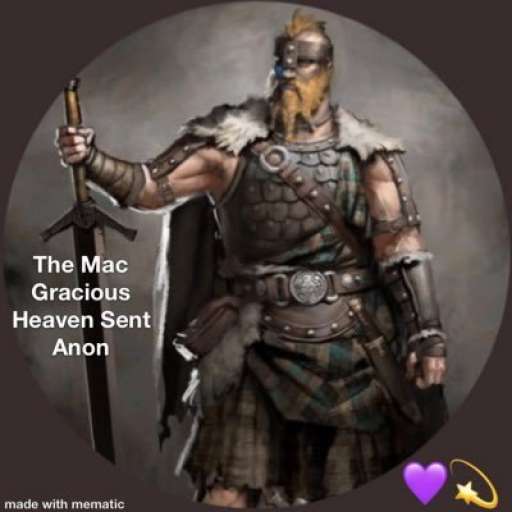
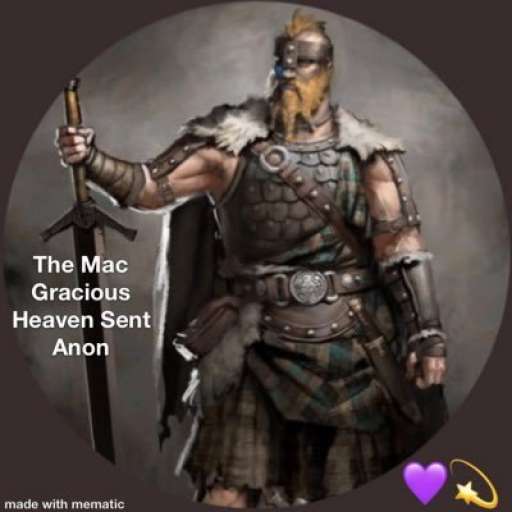
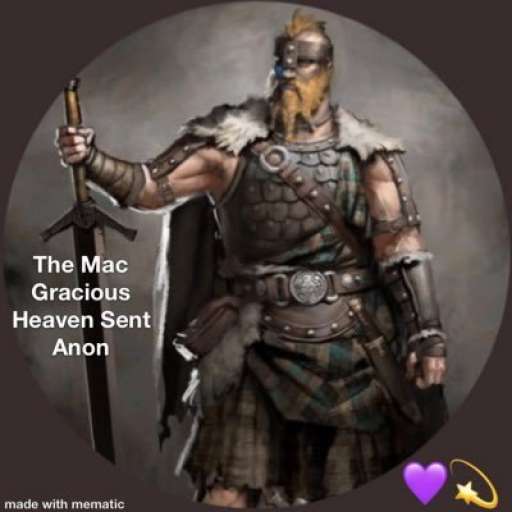
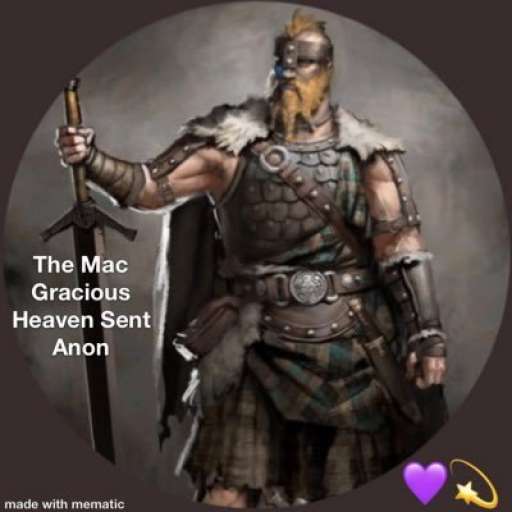
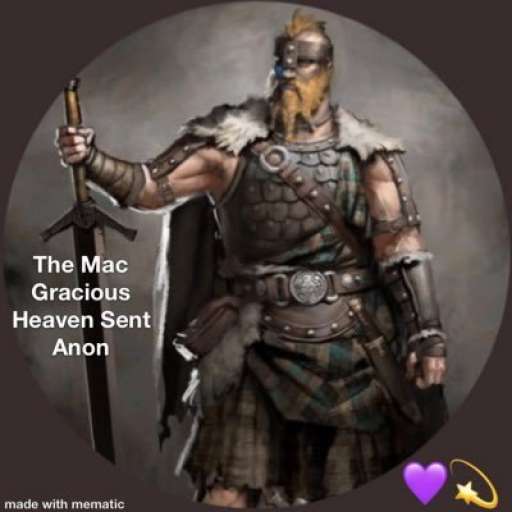
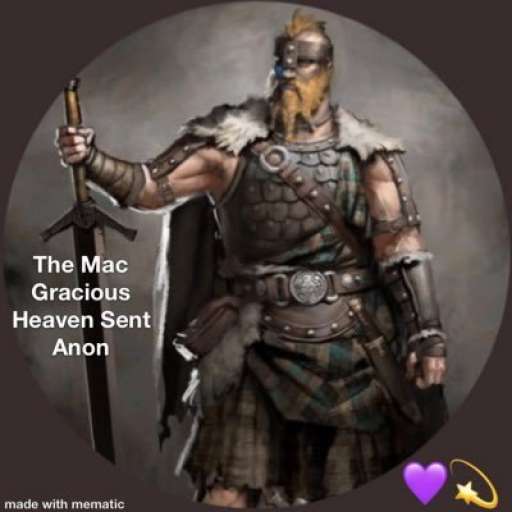
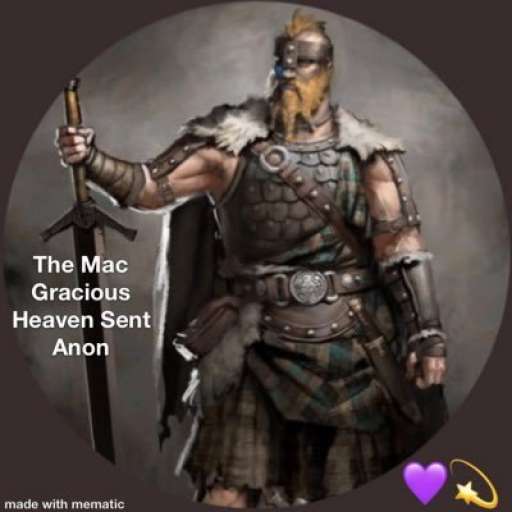
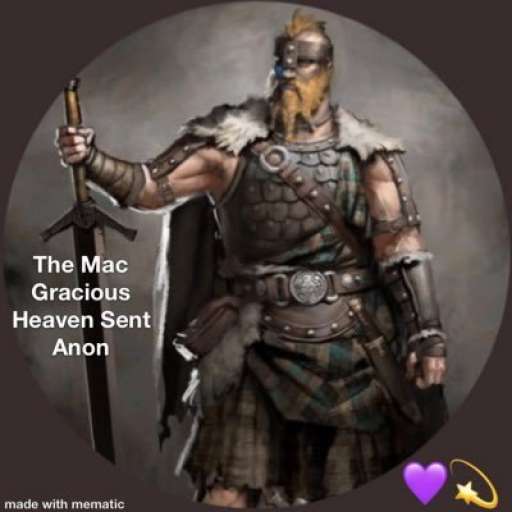
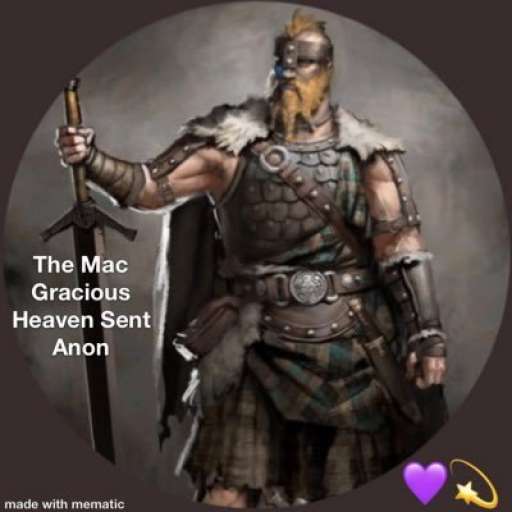
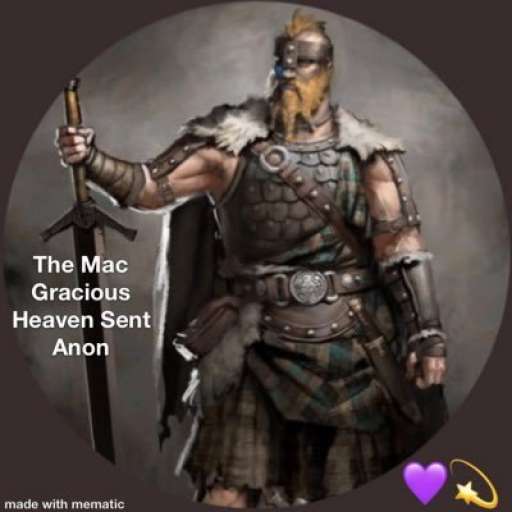
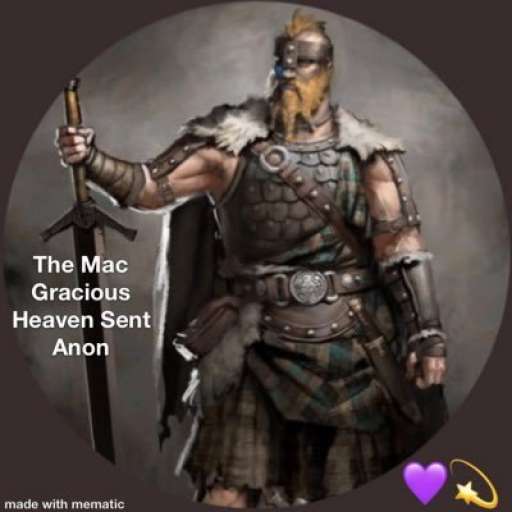
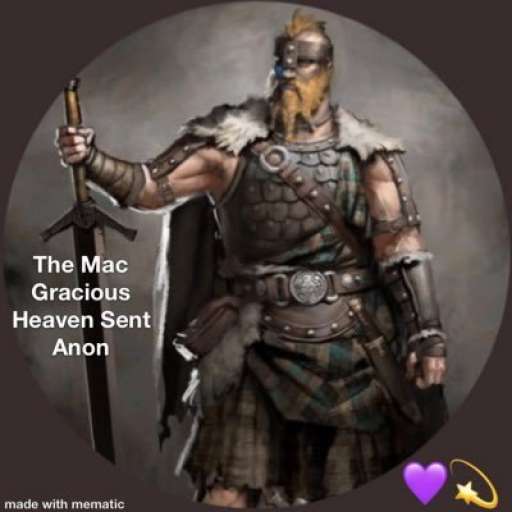
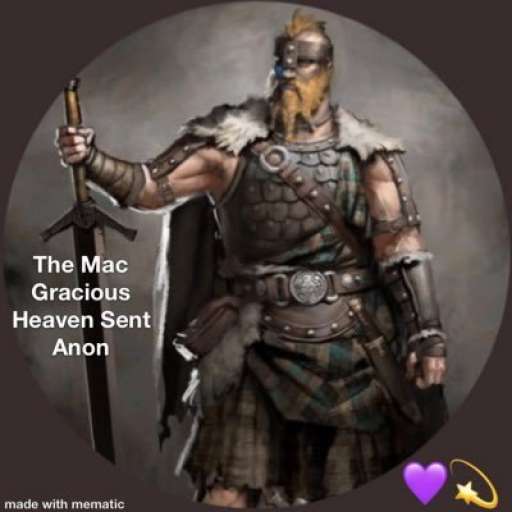
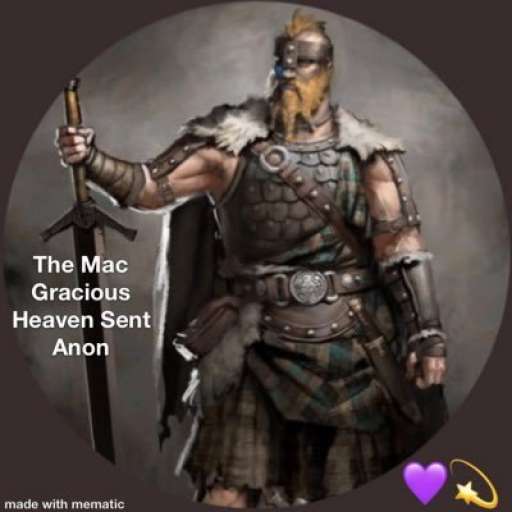
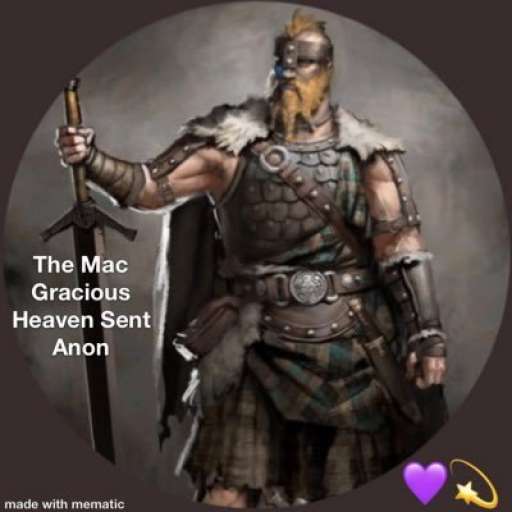
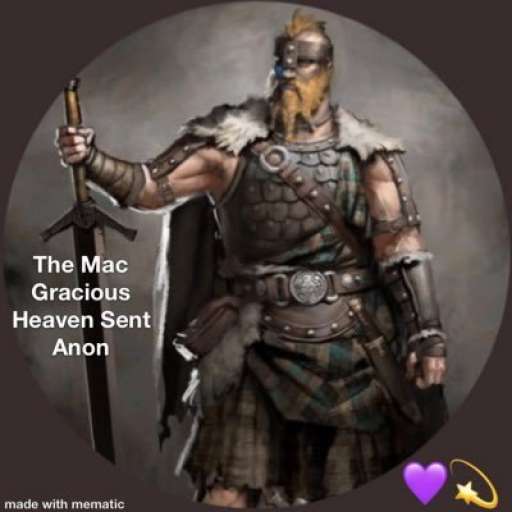
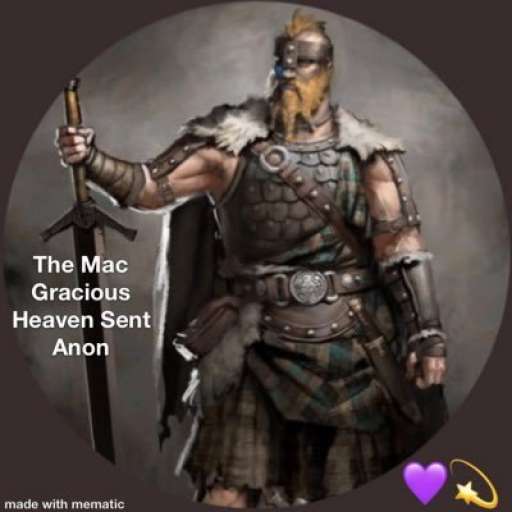
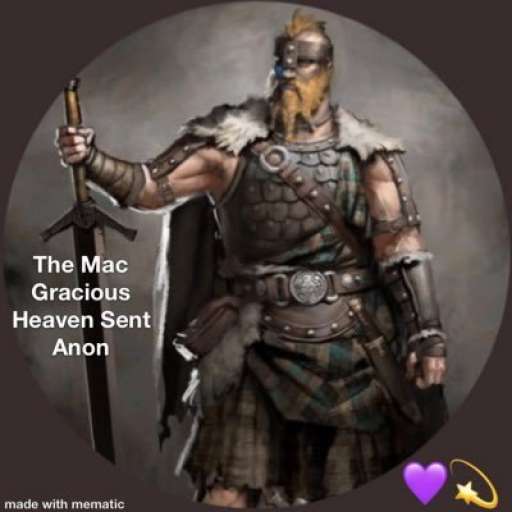
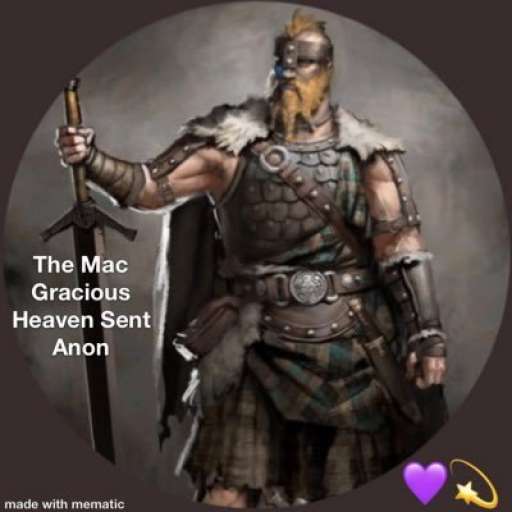
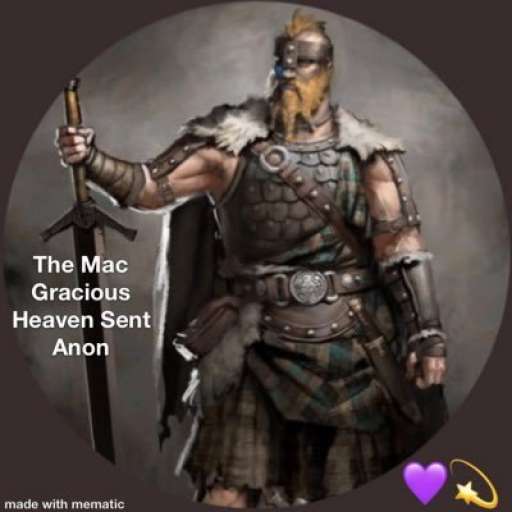
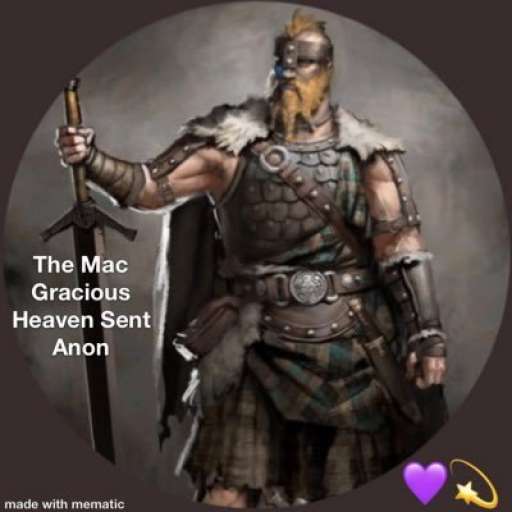
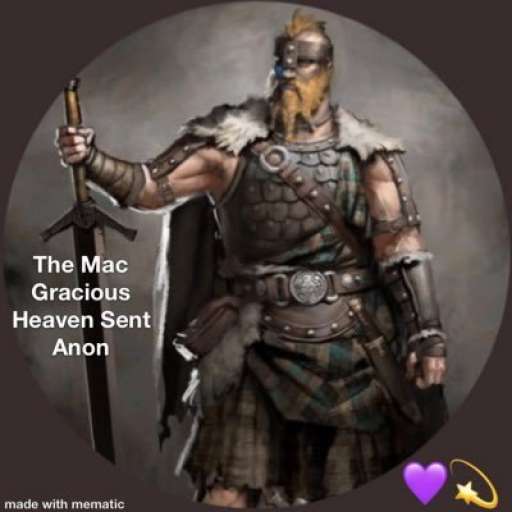
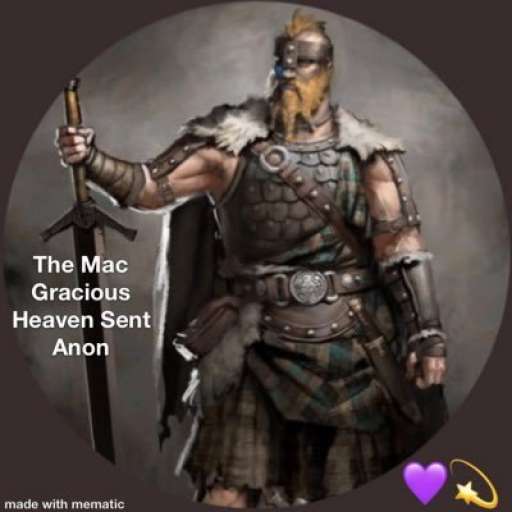
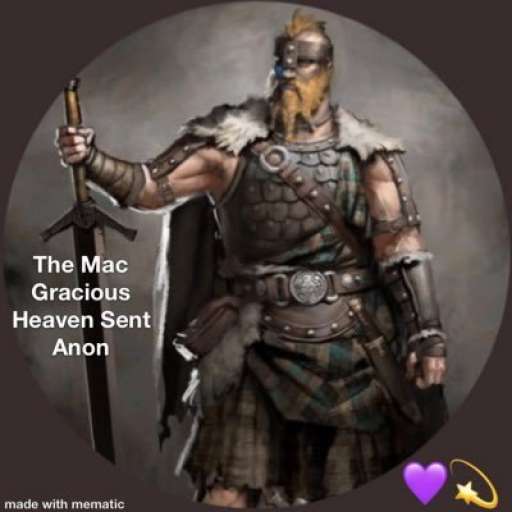
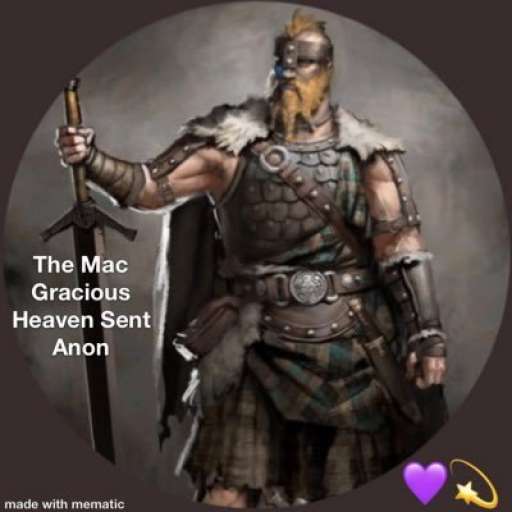
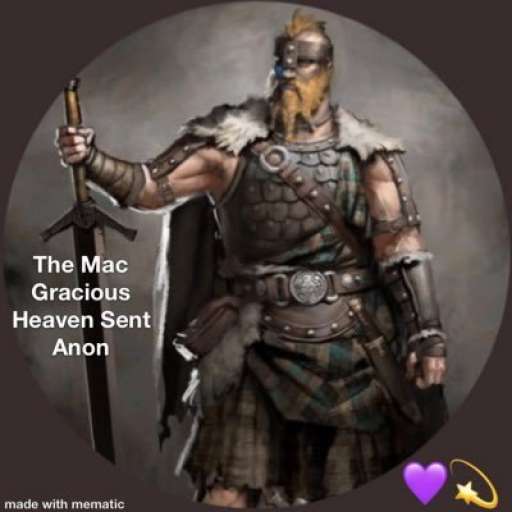
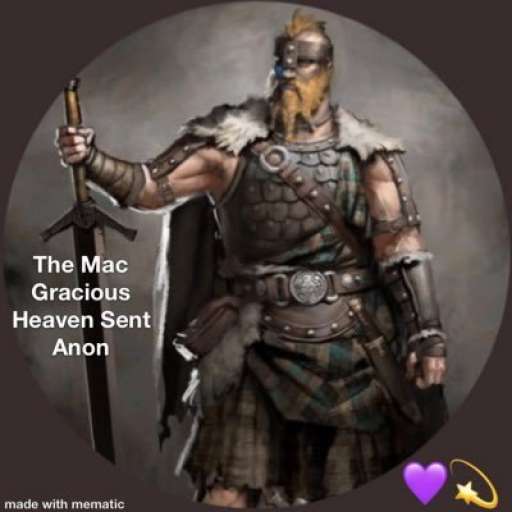
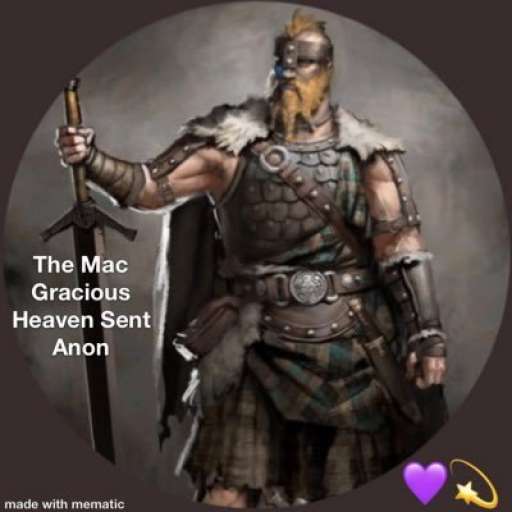
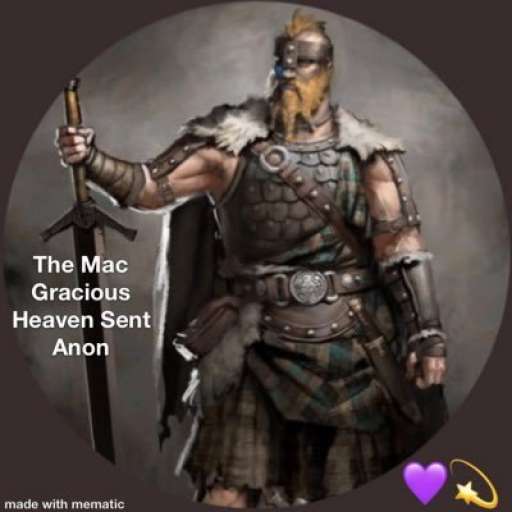
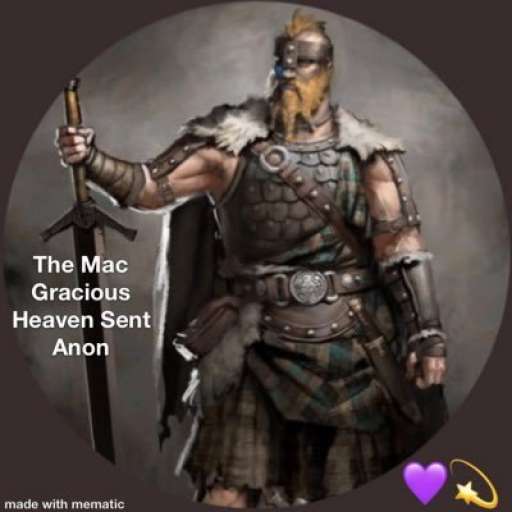
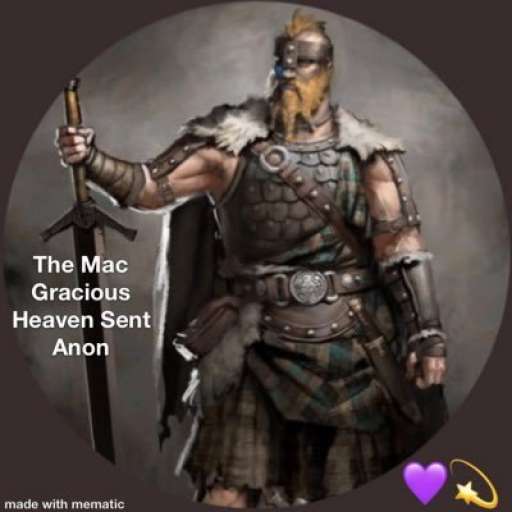
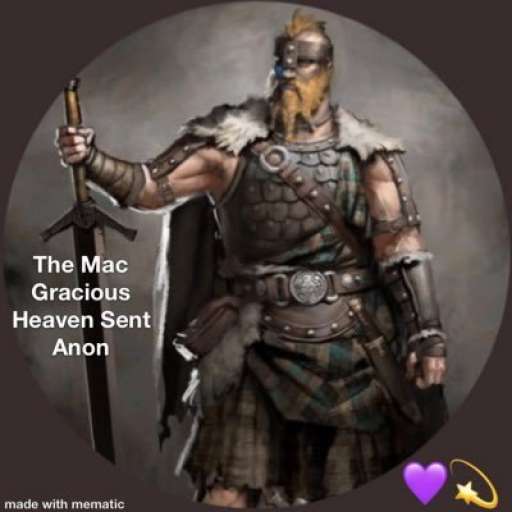
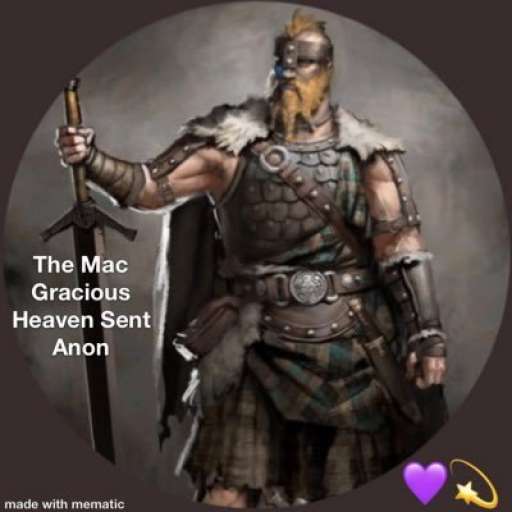
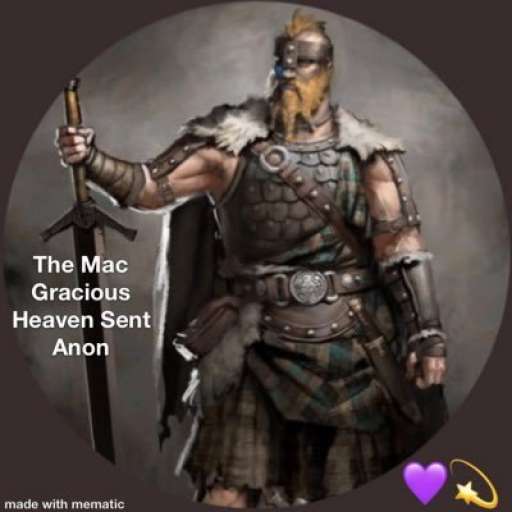
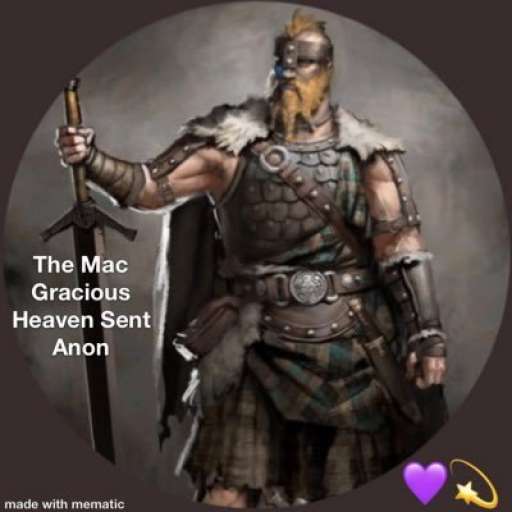
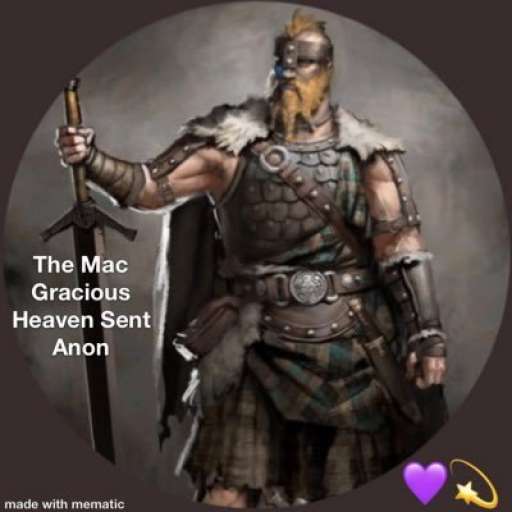
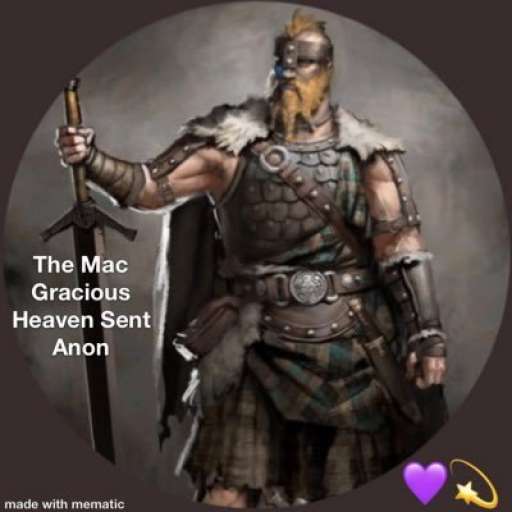
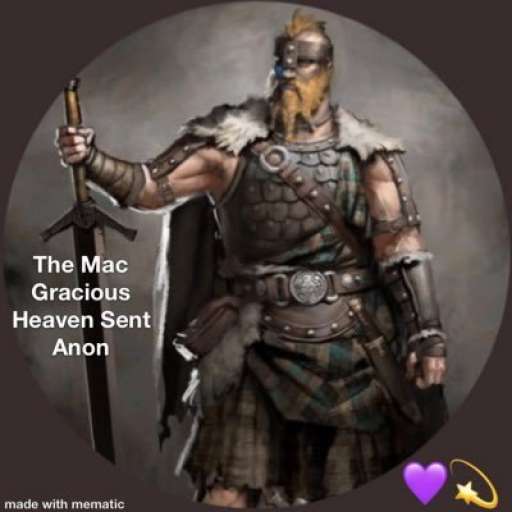
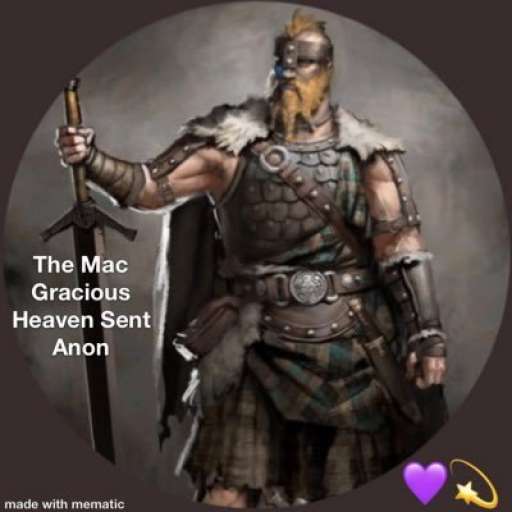
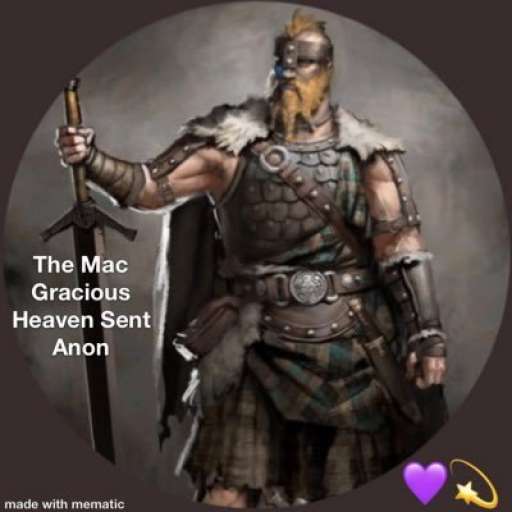
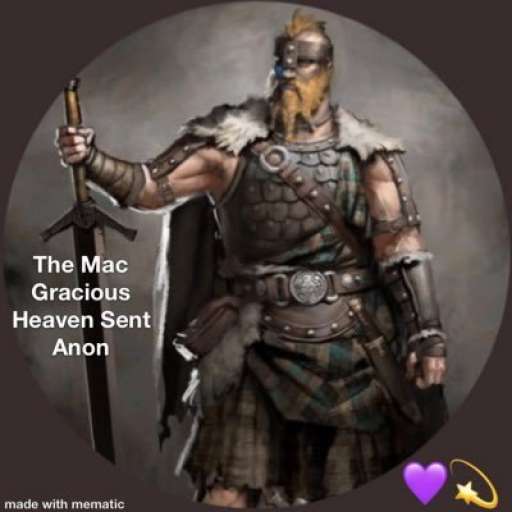
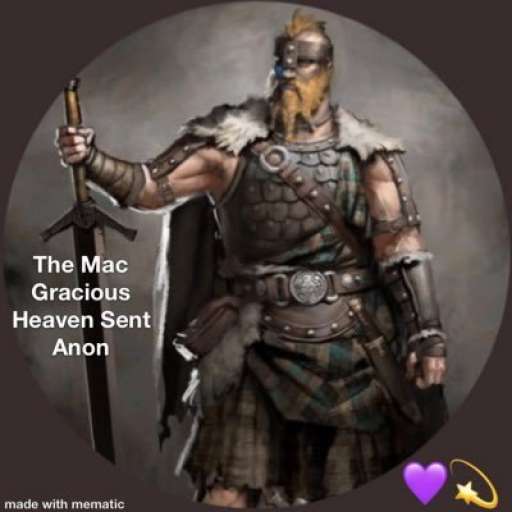
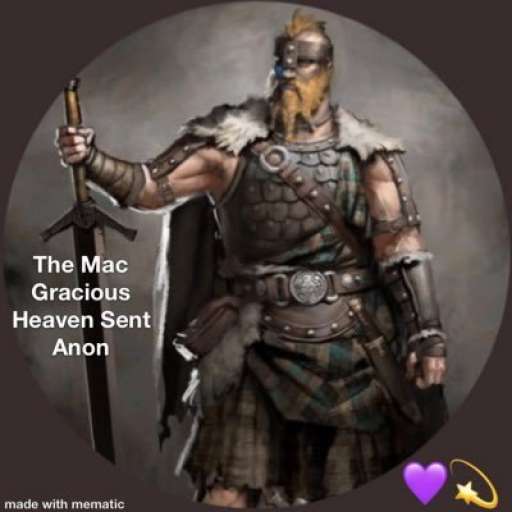
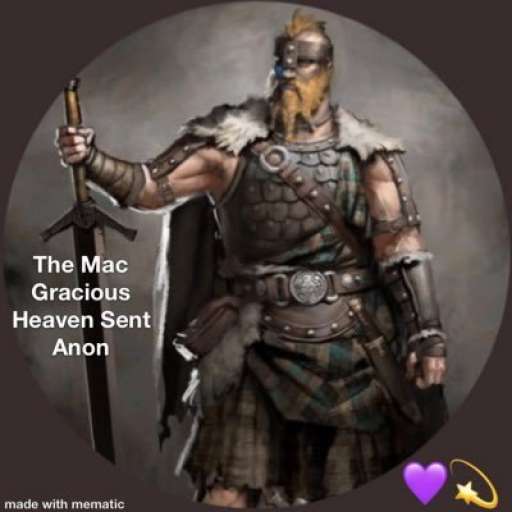
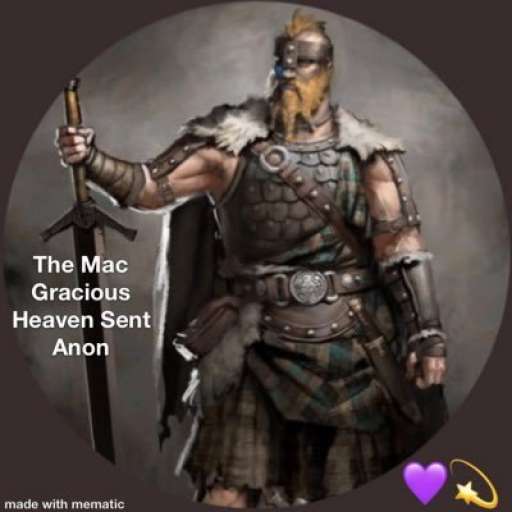
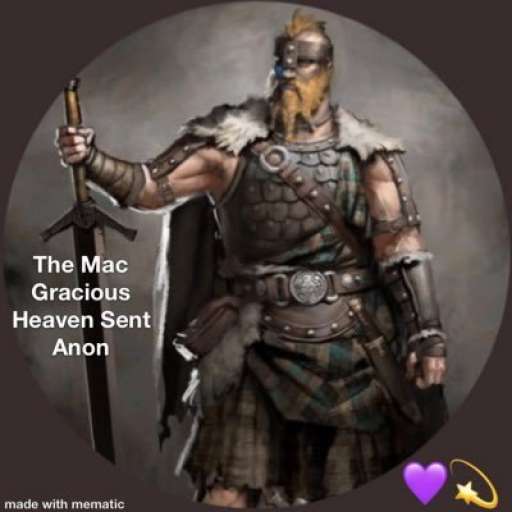
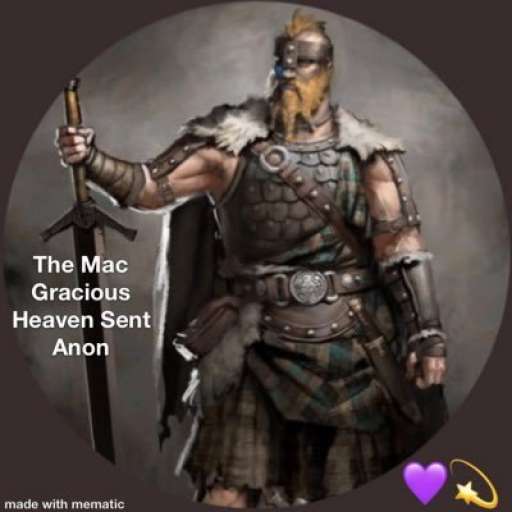
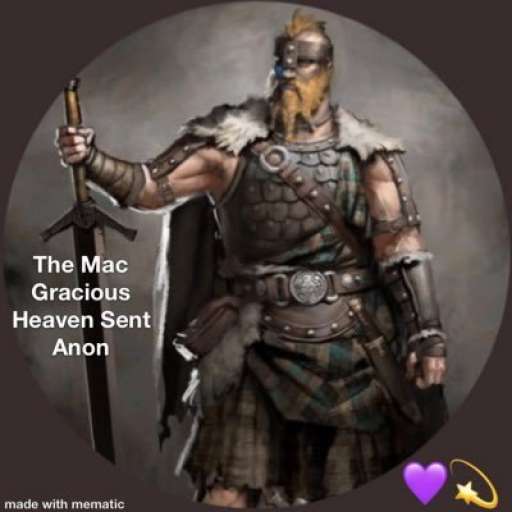
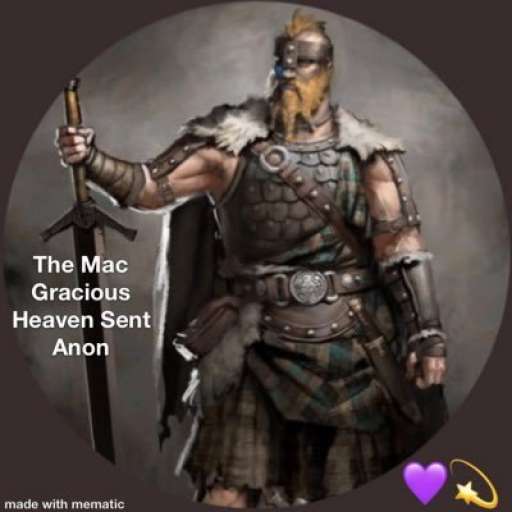
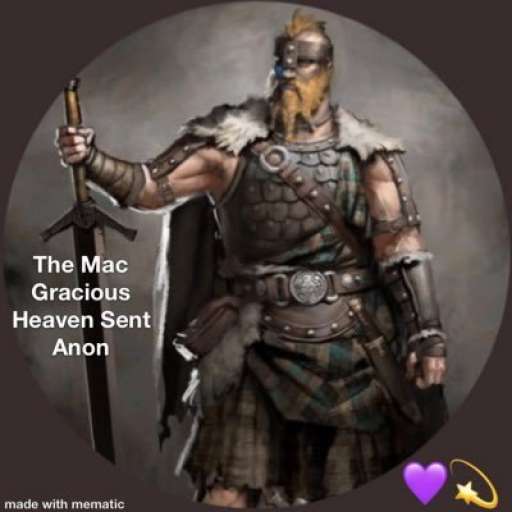
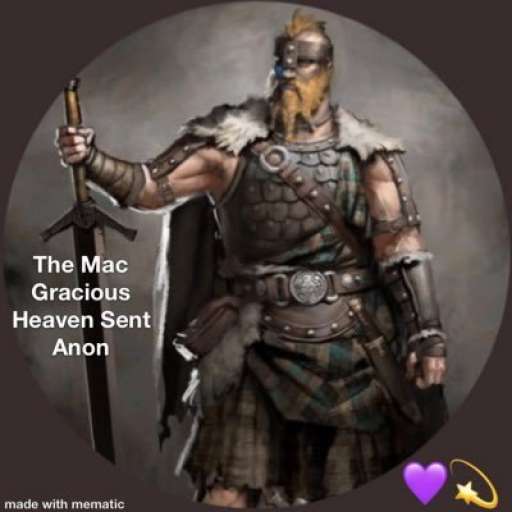
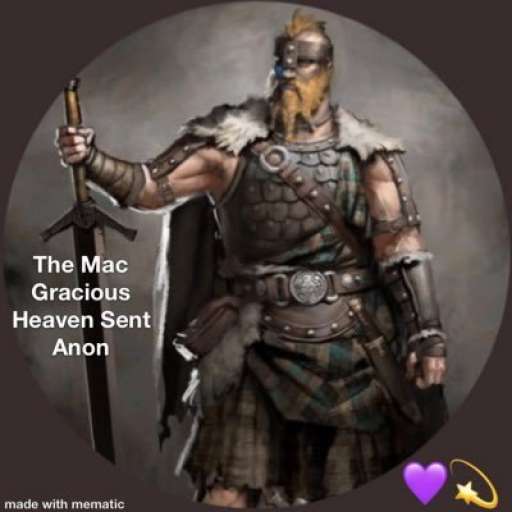
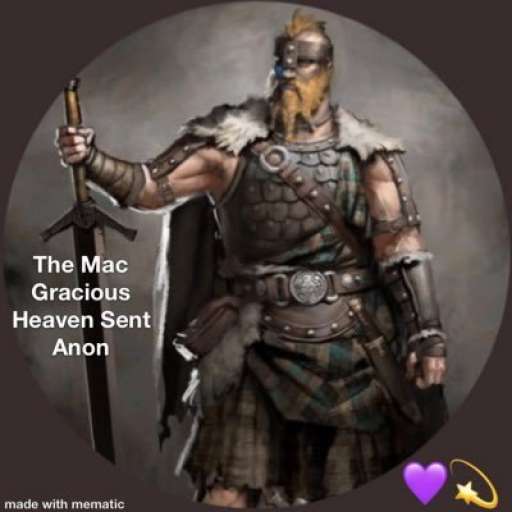
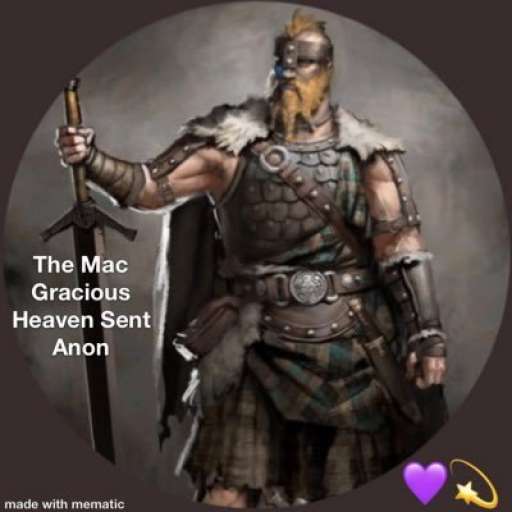
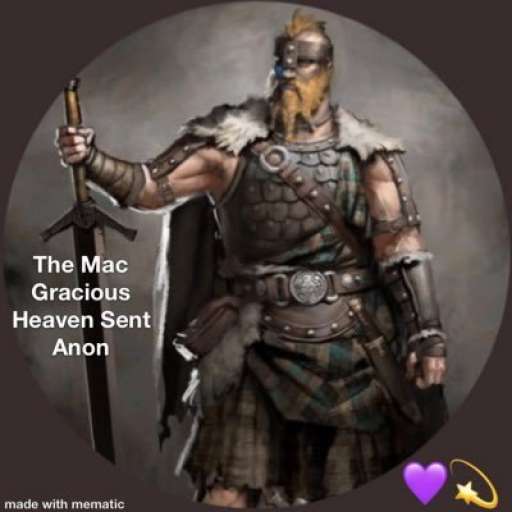
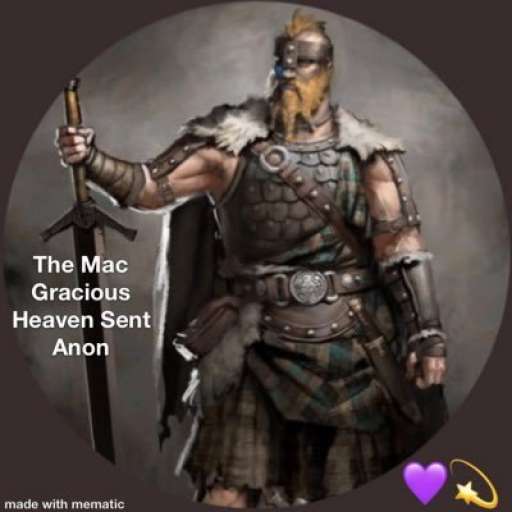
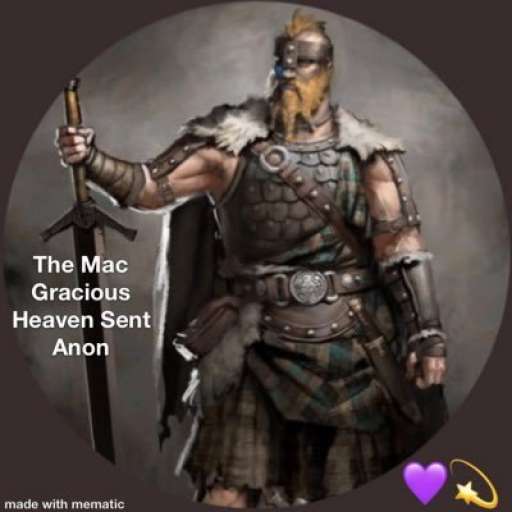
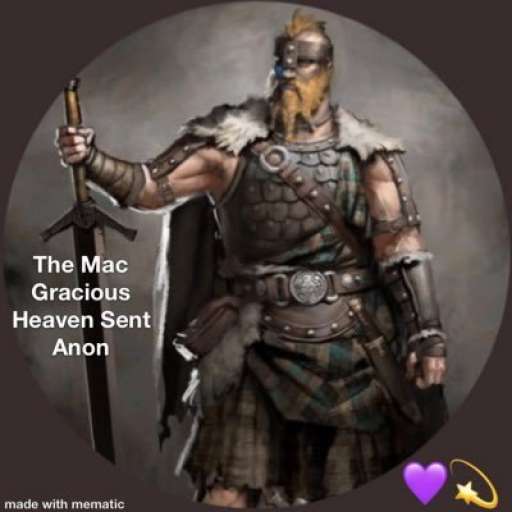
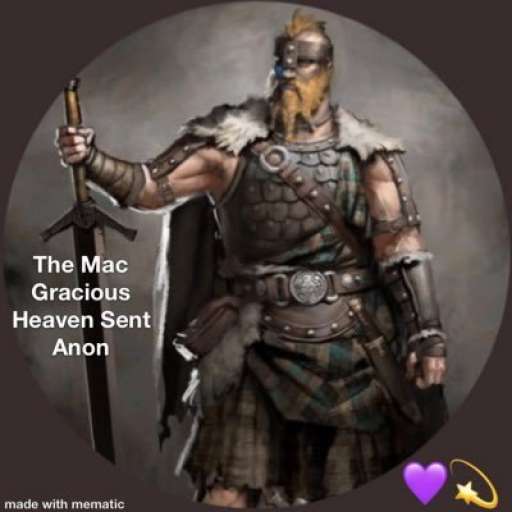
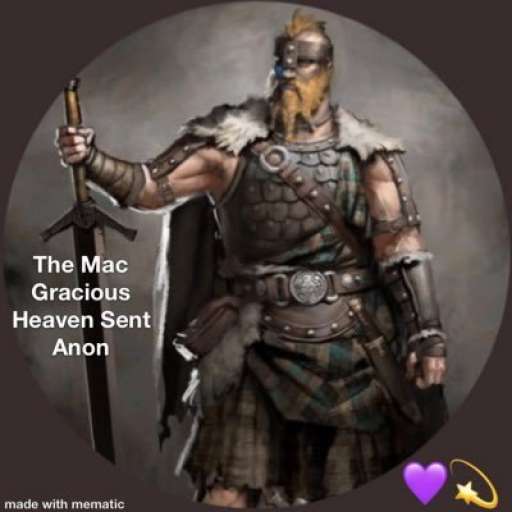
In optics, an ultrashort pulse of light is an electromagnetic pulse whose time duration is of the order of a picosecond (10−12 second) or less. Such pulses have a broadband optical spectrum, and can be created by mode-locked oscillators. They are commonly referred to as ultrafast events. Amplification of ultrashort pulses almost always requires the technique of chirped pulse amplification, in order to avoid damage to the gain medium of the amplifier.
They are characterized by a high peak intensity (or more correctly, irradiance) that usually leads to nonlinear interactions in various materials, including air. These processes are studied in the field of nonlinear optics.
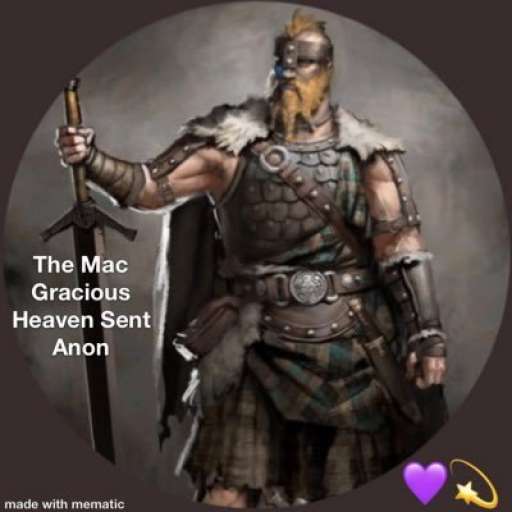
In the specialized literature, "ultrashort" refers to the femtosecond (fs) and picosecond (ps) range, although such pulses no longer hold the record for the shortest pulses artificially generated. Indeed, x-ray pulses with durations on the attosecond time scale have been reported.
The 1999 Nobel Prize in Chemistry was awarded to Ahmed H. Zewail, for the use of ultrashort pulses to observe chemical reactions at the timescales on which they occur, opening up the field of femtochemistry.
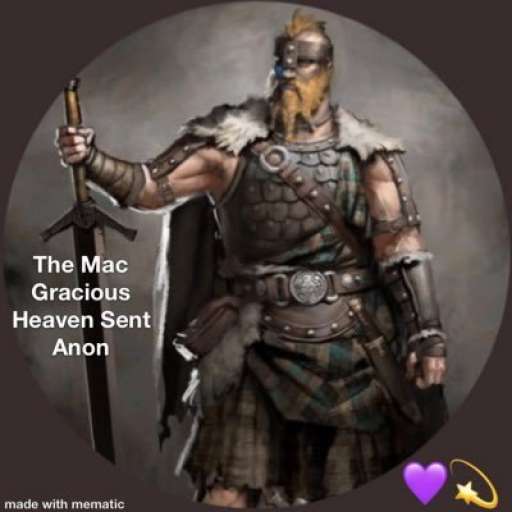
There is no standard definition of ultrashort pulse. Usually the attribute 'ultrashort' applies to pulses with a duration of a few tens of femtoseconds, but in a larger sense any pulse which lasts less than a few picoseconds can be considered ultrashort. The distinction between "Ultrashort" and "Ultrafast" is necessary as the speed at which the pulse propagates is a function of the index of refraction of the medium through which it travels, whereas "Ultrashort" refers to the temporal width of the pulse wavepacket.
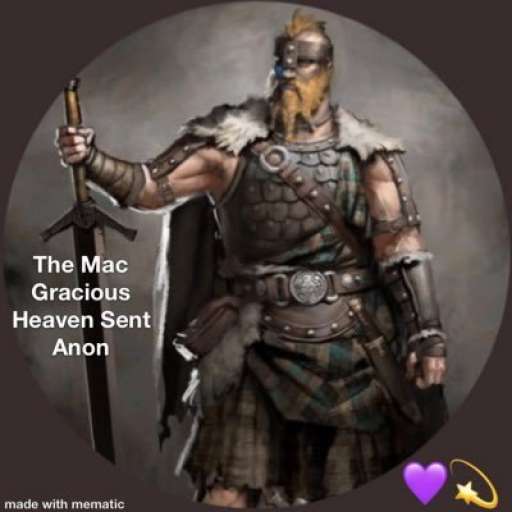
A common example is a chirped Gaussian pulse, a wave whose field amplitude follows a Gaussian envelope and whose instantaneous phase has a frequency sweep.
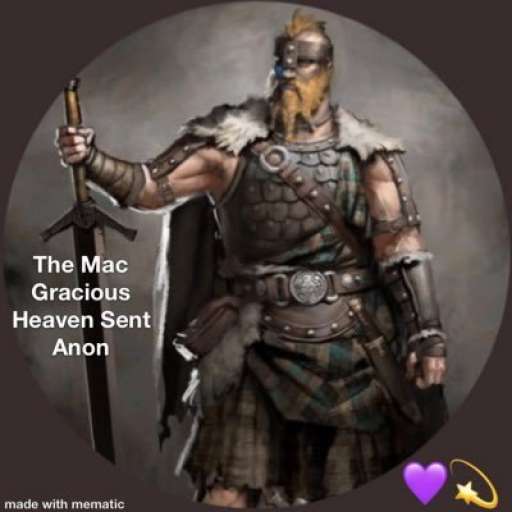
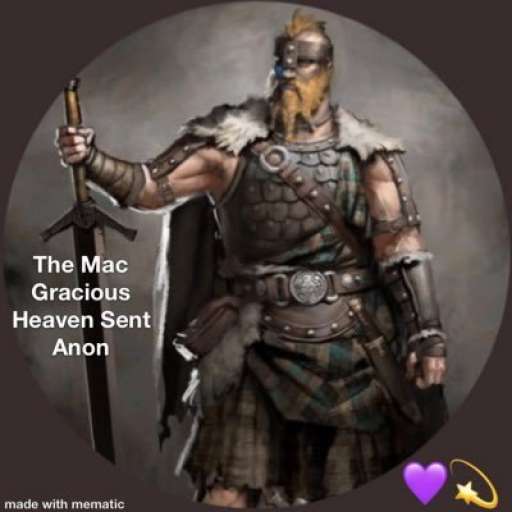
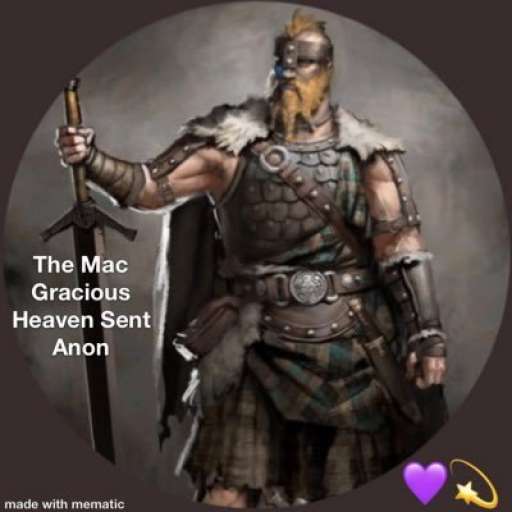
High intensity ultrashort pulse lasers can create ATI features with 20 or more peaks.[5] The photoelectron spectrum of electron energies is continuous since actual light sources contain a spread of energies.
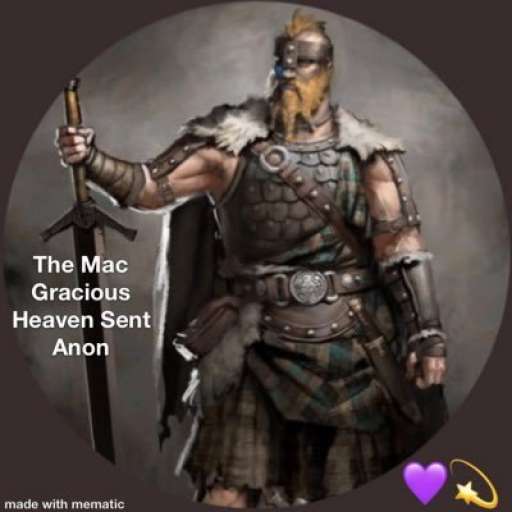
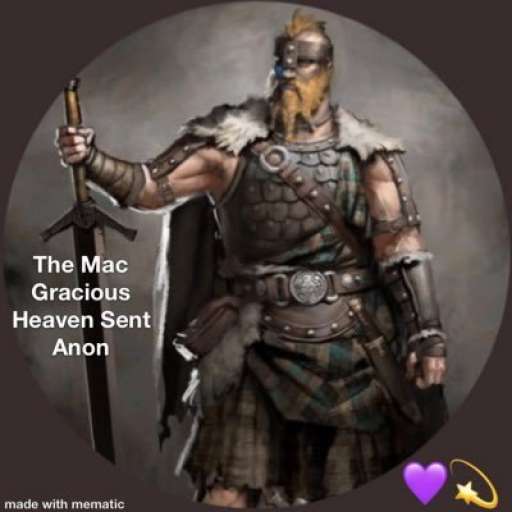
Photoionization is the physical process in which an ion is formed from the interaction of a photon with an atom or molecule.
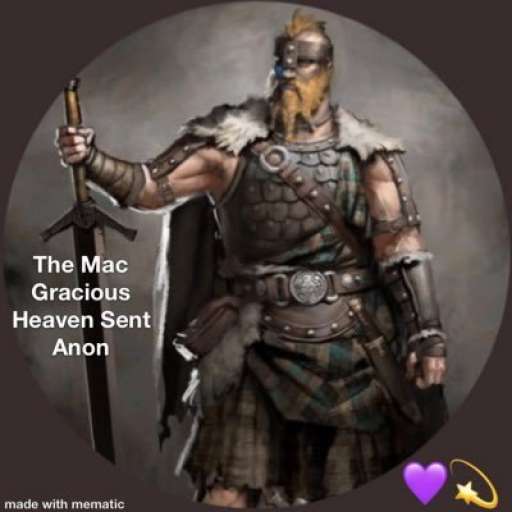
realization
/riːəlʌɪˈzeɪʃn/
noun: realization; noun: realisation
1.
an act of becoming fully aware of something as a fact.
"a growing realization of the need to create common economic structures"
awareness
understanding
comprehension
consciousness
apprehension
cognizance
appreciation
recognition
perception
discernment
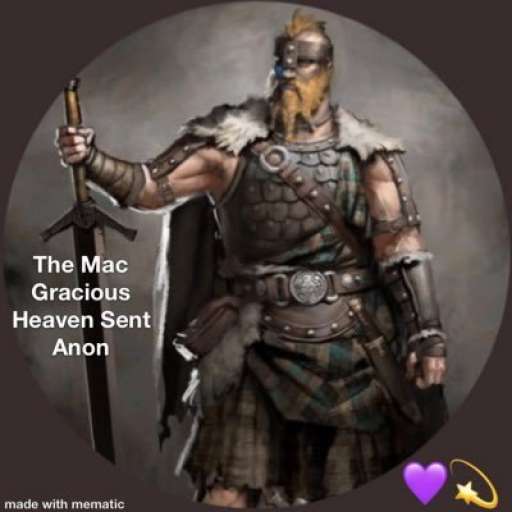
the achievement of something desired or anticipated.
actualization
fulfilment
achievement
accomplishment
attainment
bringing to fruition
bringing into being
consummation
effecting
effectuation
reification
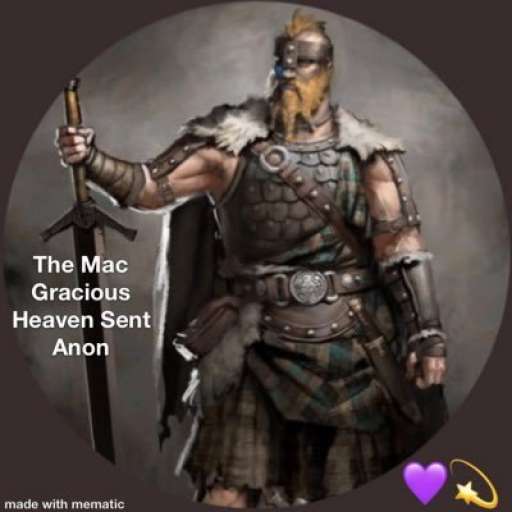
the making or being made real of something imagined, planned, etc. the result of such a process: The new church was the realization of a ten-year dream. the act of realizing or the state of being realized. an instance or result of realizing.
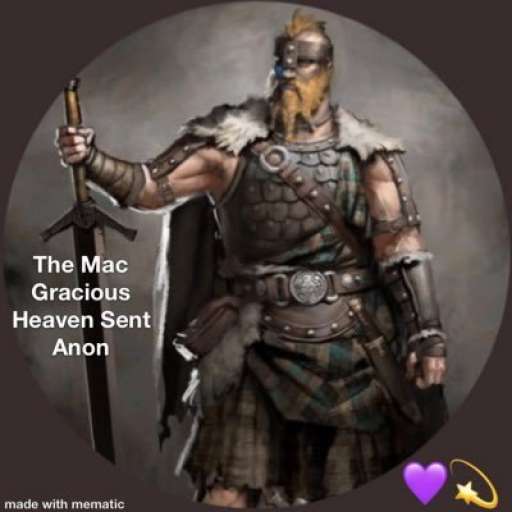
Not every interaction between a photon and an atom, or molecule, will result in photoionization. The probability of photoionization is related to the photoionization cross section of the species, which depends on the energy of the photon (proportional to its wavenumber) and the species being considered. In the case of molecules, the photoionization cross-section can be estimated by examination of Franck-Condon factors between a ground-state molecule and the target ion. For photon energies below the ionization threshold, the photoionization cross-section is near zero. But with the development of pulsed lasers it has become possible to create extremely intense, coherent light where multi-photon ionization may occur. At even higher intensities (around 1015 – 1016 W/cm2 of infrared or visible light), non-perturbative phenomena such as barrier suppression ionization[3] and rescattering ionization[4] are observed.
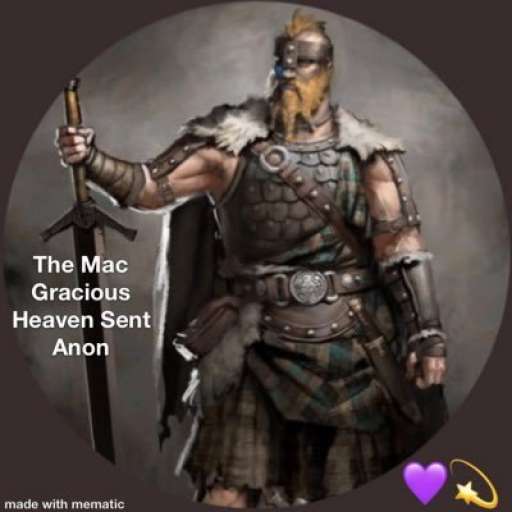
Resonance-enhanced multiphoton ionization (REMPI) is a technique applied to the spectroscopy of atoms and small molecules in which a tunable laser can be used to access an excited intermediate state.
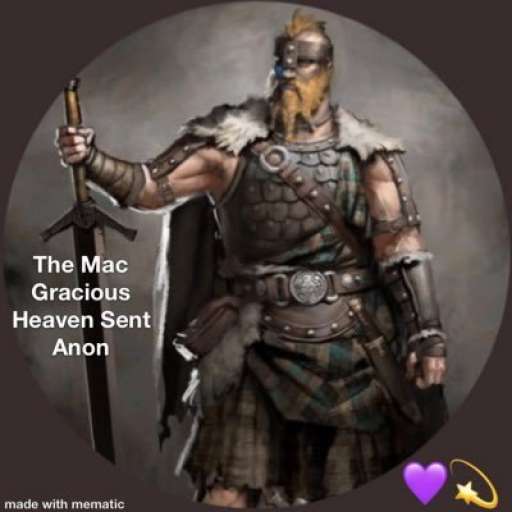
A simple example of this concept comes by considering the hydrogen atom.
The ground state of the hydrogen atom corresponds to having the atom's single electron in the lowest possible orbital (that is, the spherically symmetric "1s" wave function, which, so far, has demonstrated to have the lowest possible quantum numbers). By giving the atom additional energy (for example, by the absorption of a photon of an appropriate energy), the electron is able to move into an excited state (one with one or more quantum numbers greater than the minimum possible). If the photon has too much energy, the electron will cease to be bound to the atom, and the atom will become ionized.
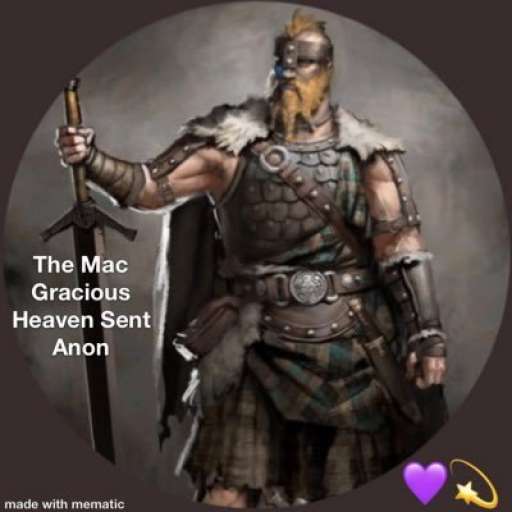
After excitation the atom may return to the ground state or a lower excited state, by emitting a photon with a characteristic energy. Emission of photons from atoms in various excited states leads to an electromagnetic spectrum showing a series of characteristic emission lines (including, in the case of the hydrogen atom, the Lyman, Balmer, Paschen and Brackett series.)
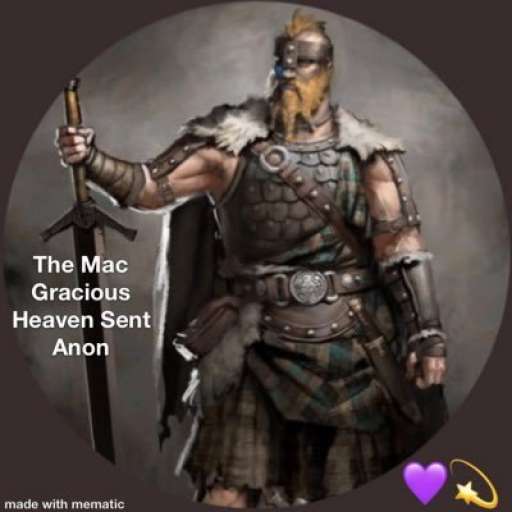
The excitation of a system (an atom or molecule) from one excited state to a higher energy excited state with the absorption of a photon is called excited state absorption (ESA). Excited state absorption is possible only when an electron has been already excited from the ground state to a lower excited state. The excited state absorption is usually an undesired effect, but it can be useful in upconversion pumping.[8] Excited state absorption measurements are done using pump-probe techniques such as flash photolysis. However, it is not easy to measure them compared to ground-state absorption and in some cases complete bleaching of the ground state is required to measure excited state absorption.[9]
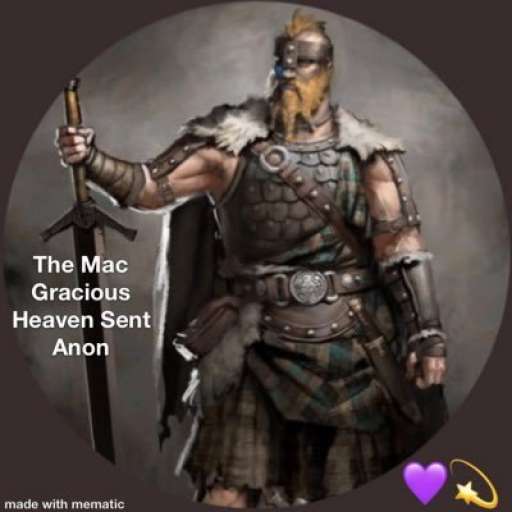
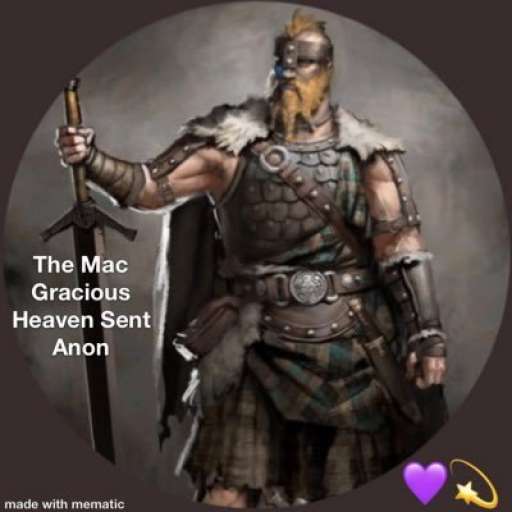
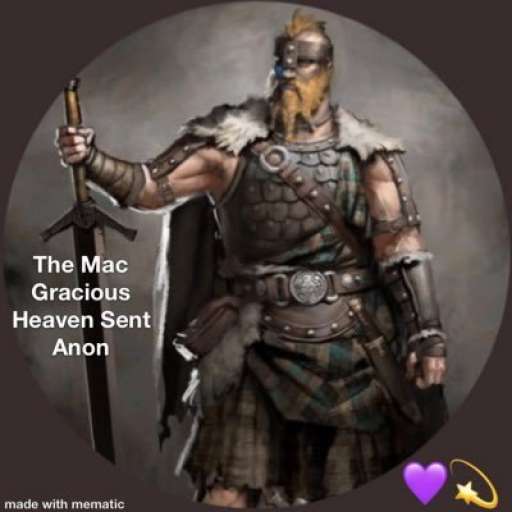
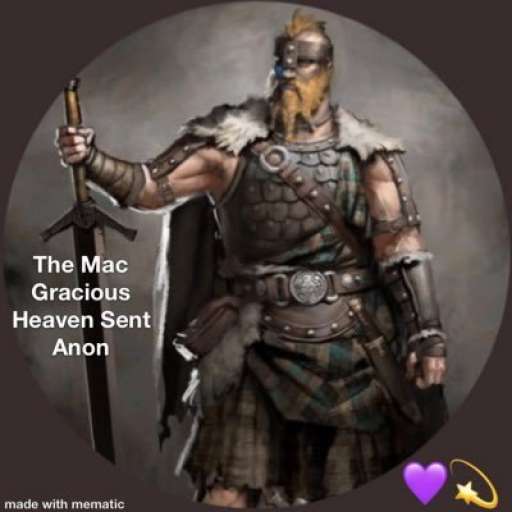
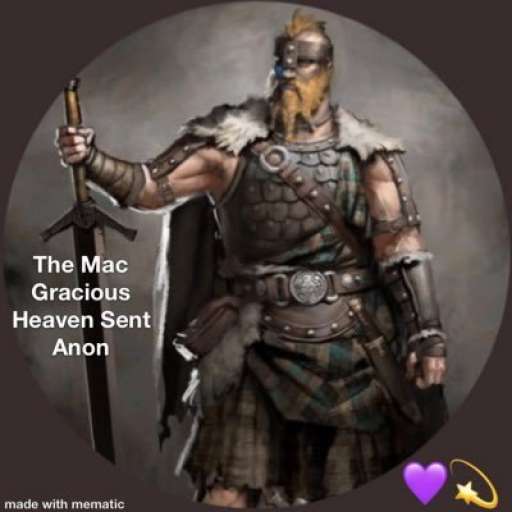
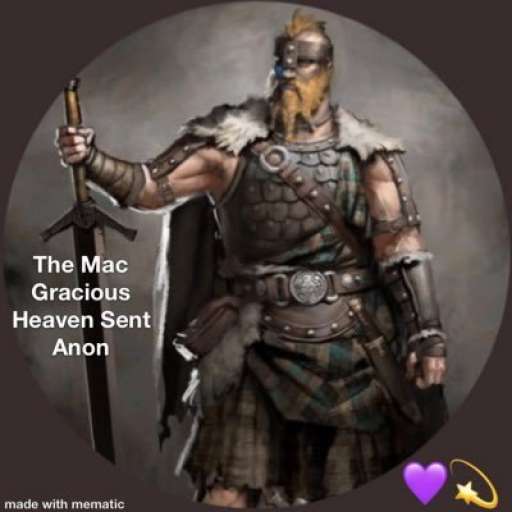
An emerging class of inorganic optical reporters are near-infrared (NIR) excitable lanthanide-based upconversion nanoparticles (UCNPs) with multicolor emission and long luminescence lifetimes in the range of several hundred microseconds. For the design of chemical sensors and optical probes that reveal analyte-specific changes in their spectroscopic properties, these nanomaterials must be combined with sensitive indicator dyes that change their absorption and/or fluorescence properties selectively upon interaction with their target analyte, utilizing either resonance energy transfer (RET) processes or reabsorption-related inner filter effects.
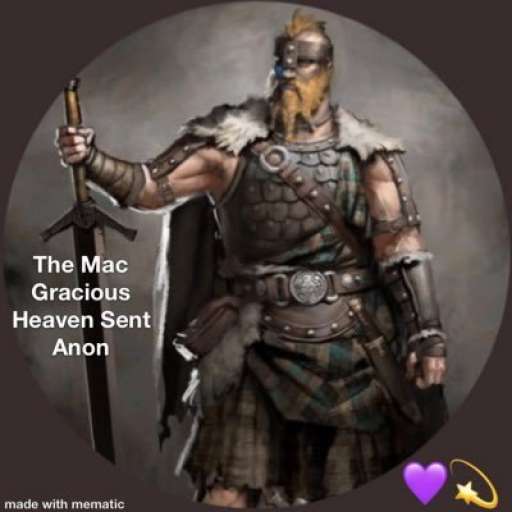
The rational development of UCNP-based nanoprobes for chemical sensing and imaging in a biological environment requires reliable methods for the surface functionalization of UCNPs, the analysis and quantification of surface groups, a high colloidal stability of UCNPs in aqueous media as well as the chemically stable attachment of the indicator molecules, and suitable instrumentation for the spectroscopic characterization of the energy-transfer systems and the derived nanosensors. These topics are highlighted in the following feature article, and examples of functionalized core–shell nanoprobes for the sensing of different biologically relevant analytes in aqueous environments will be presented. Special emphasis is placed on the intracellular sensing of pH
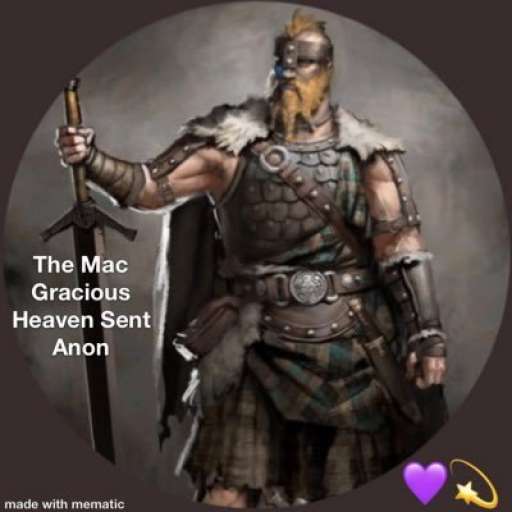
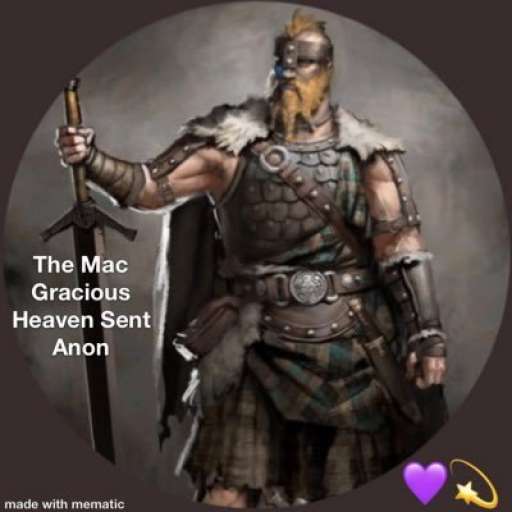
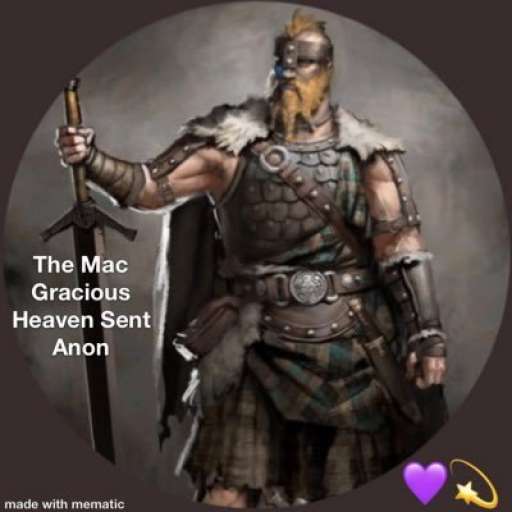
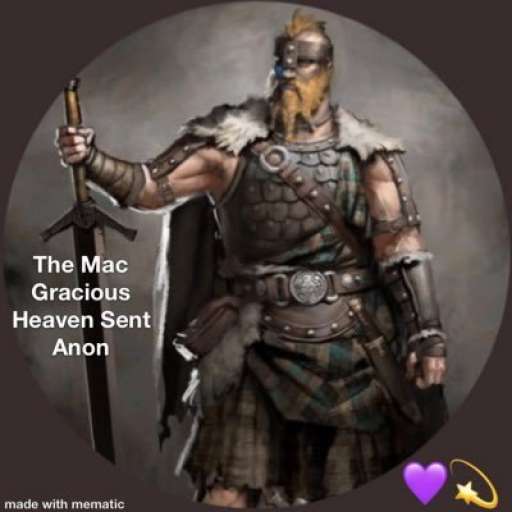
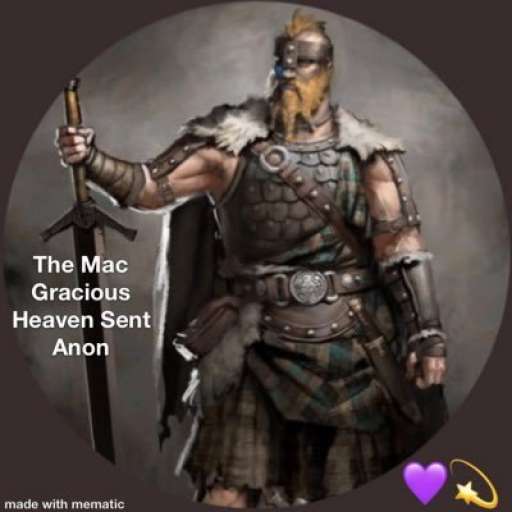
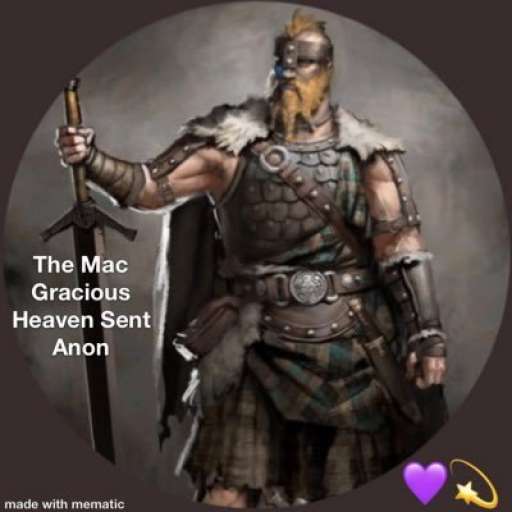
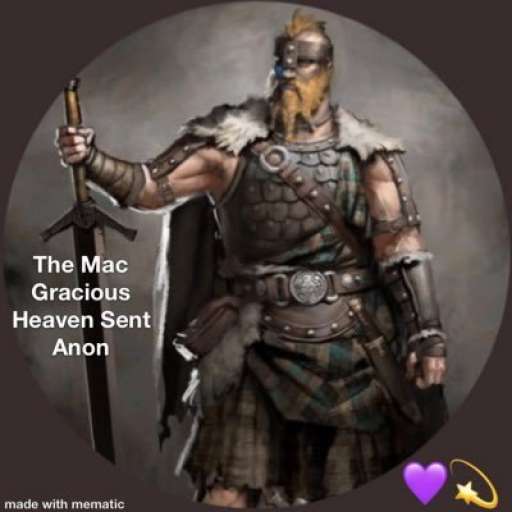
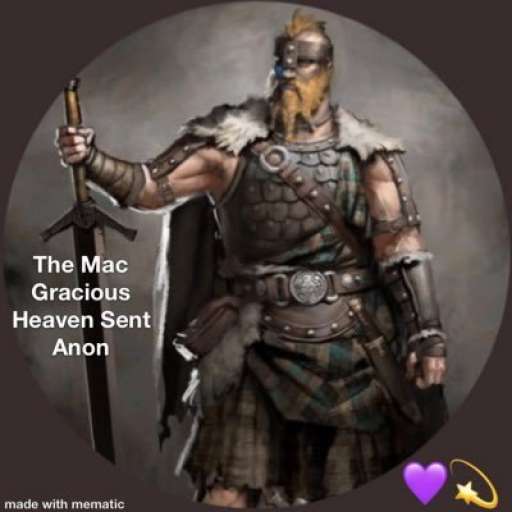
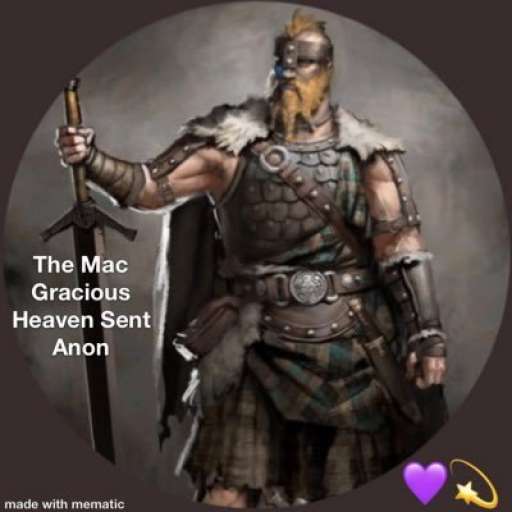
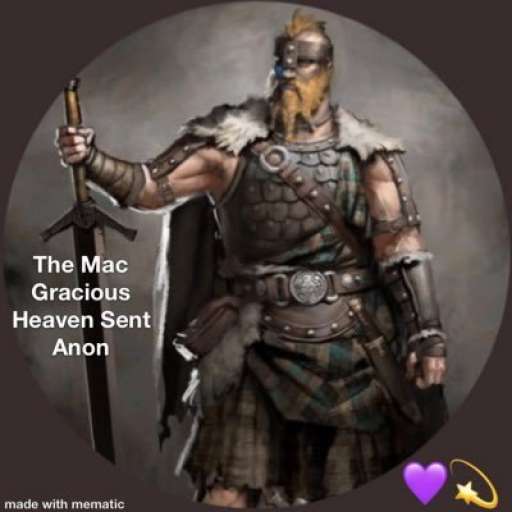
Finished.
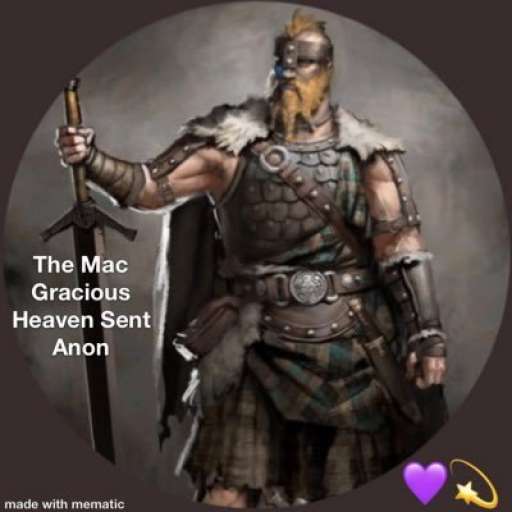
finishen
(intransitive, racing) to finish
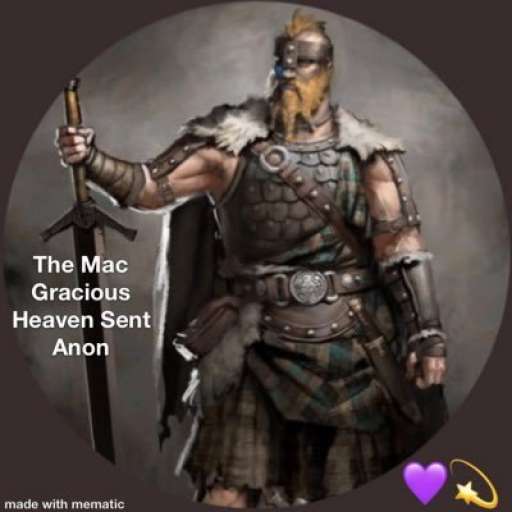
F I N I S H E N D
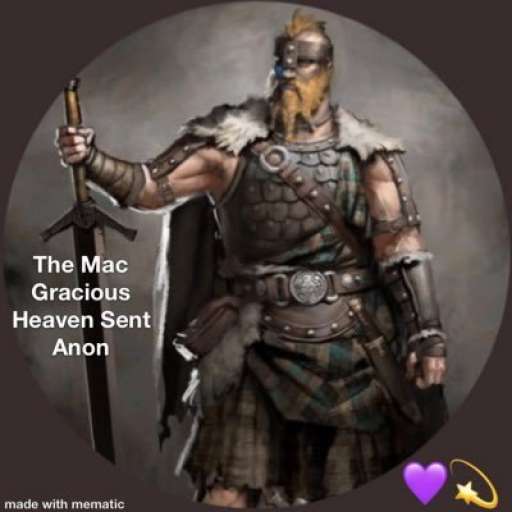
The Proto-Germanic meaning of *ǥuđán and its etymology is uncertain. It is generally agreed that it derives from a Proto-Indo-European neuter passive perfect participle *ǵʰu-tó-m. This form within (late) Proto-Indo-European itself was possibly ambiguous, and thought to derive from a root *ǵʰeu̯- "to pour, libate" (the idea survives in the Dutch word, 'Giet', meaning, to pour) (Sanskrit huta, see hotṛ), or from a root *ǵʰau̯- (*ǵʰeu̯h2-) "to call, to invoke" (Sanskrit hūta). Sanskrit hutá = "having been sacrificed", from the verb root hu = "sacrifice", but a slight shift in translation gives the meaning "one to whom sacrifices are made."
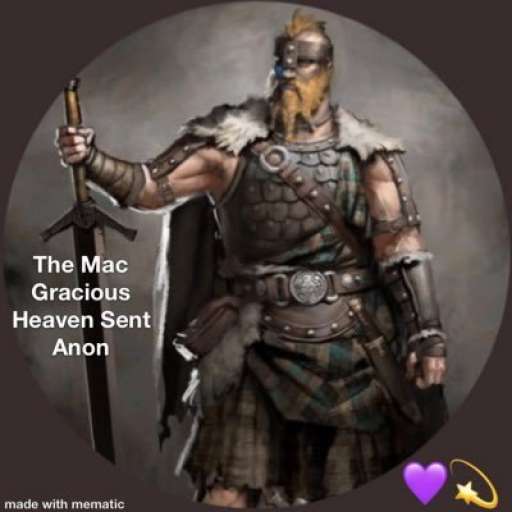
The English word god comes from the Old English god, which itself is derived from the Proto-Germanic *ǥuđán. Its cognates in other Germanic languages include guþ, gudis (both Gothic), guð (Old Norse), god (Old Saxon, Old Frisian, and Old Dutch), and got (Old High German).
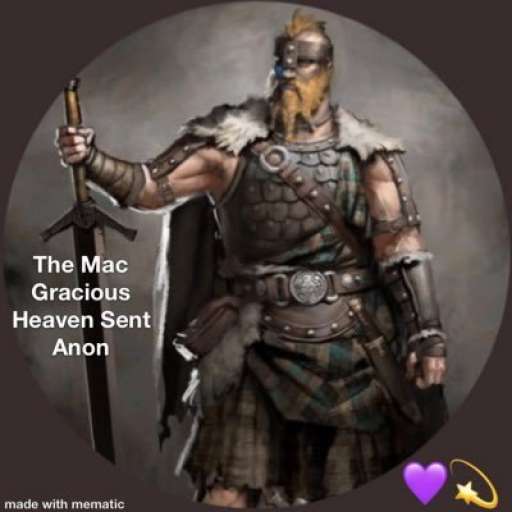
The earliest uses of the word God in Germanic writing is often cited to be in the Gothic Bible or Wulfila Bible, which is the Christian Bible as translated by Ulfilas into the Gothic language spoken by the Eastern Germanic, or Gothic, tribes. The oldest parts of the Gothic Bible, contained in the Codex Argenteus, are estimated to be from the fourth century. During the fourth century, the Goths were converted to Christianity, largely through the efforts of Bishop Ulfilas, who translated the Bible into the Gothic language in Nicopolis ad Istrum in today's northern Bulgaria. The words guda and guþ were used for God in the Gothic Bible.
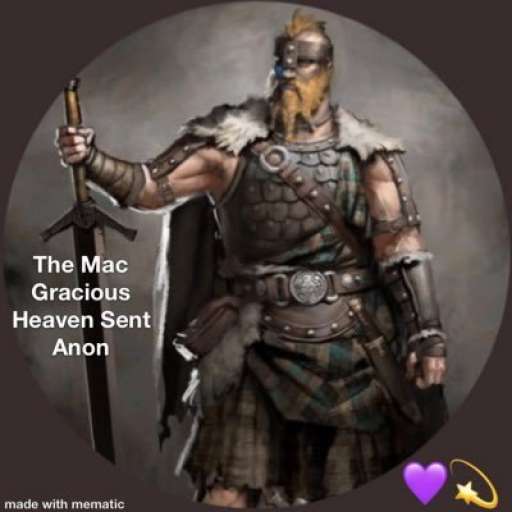
Bible
/ˈbʌɪb(ə)l/
noun
singular proper noun: Bible; noun: the Bible; noun: bible
1.
the Christian scriptures, consisting of the Old and New Testaments.
"verses from the Bible"
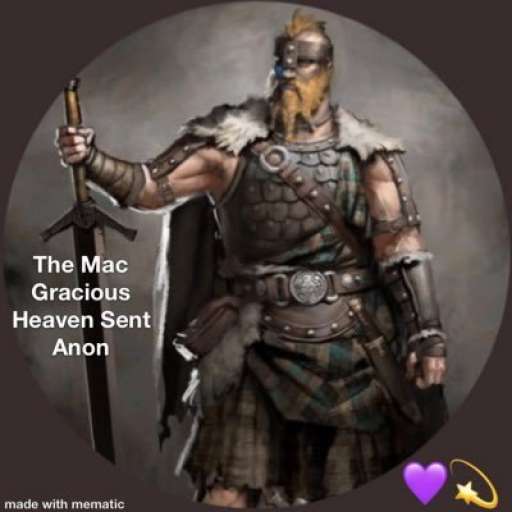
The difference between New and Old. When used as nouns, new means things that are new, whereas old means people who are old. When used as adjectives, new means recently made, or created, whereas old means of an object, concept, relationship, etc., having existed for a relatively long period of time.
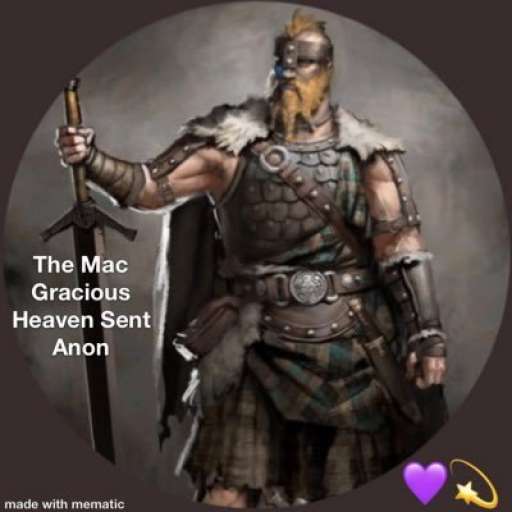
A time-variant system is a system whose output response depends on moment of observation as well as moment of input signal application.[1] In other words, a time delay or time advance of input not only shifts the output signal in time but also changes other parameters and behavior. Time variant systems respond differently to the same input at different times. The opposite is true for time invariant systems (TIV).
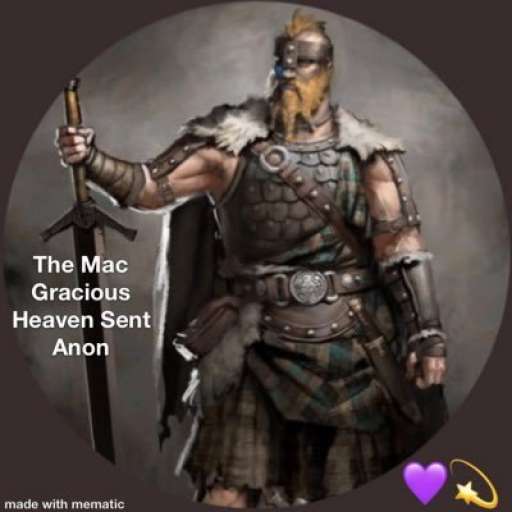
Sine wave example
We can observe the phase shifting effect thru a sine wave. ... Time delay shifts all frequencies by the same amount of time, whereas phase shift delays some frequencies longer than others. In fact, an all-pass filters center frequency is defined at the frequency at which the phase shift is 90 degrees
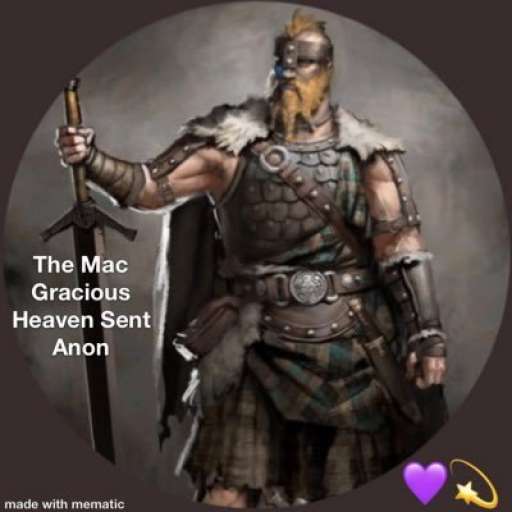
God standeth in the congregation of the mighty; he judgeth among the gods.
How long will ye judge unjustly, and accept the persons of the wicked? Selah.
Defend the poor and fatherless: do justice to the afflicted and needy.
Deliver the poor and needy: rid them out of the hand of the wicked.
They know not, neither will they understand; they walk on in darkness: all the foundations of the earth are out of course.
I have said, Ye are gods; and all of you are children of the most High.
But ye shall die like men, and fall like one of the princes.
Arise, O God, judge the earth: for thou shalt inherit all nations.
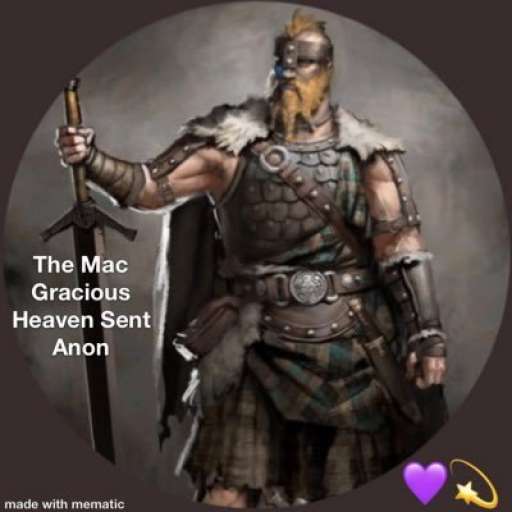
There could be delays to the deepstates plan?
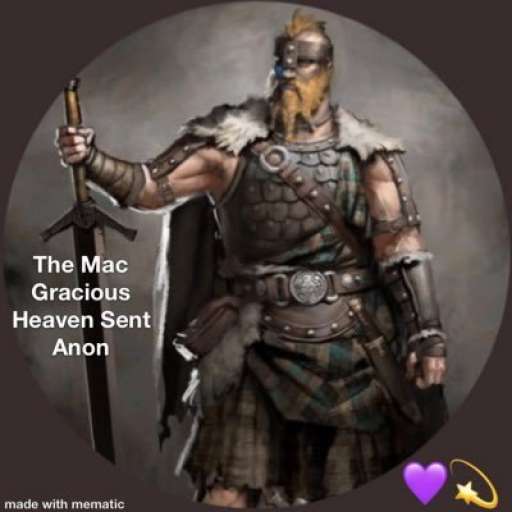
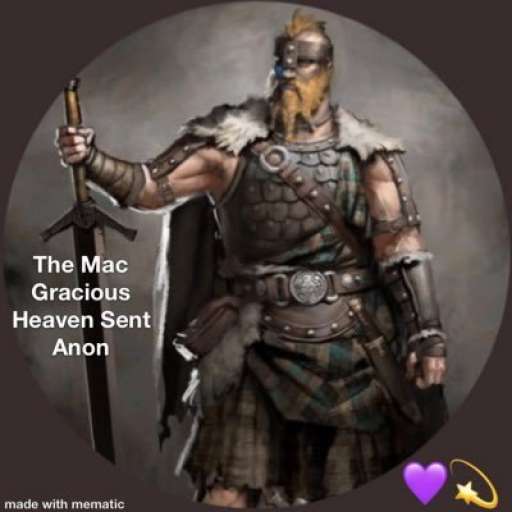
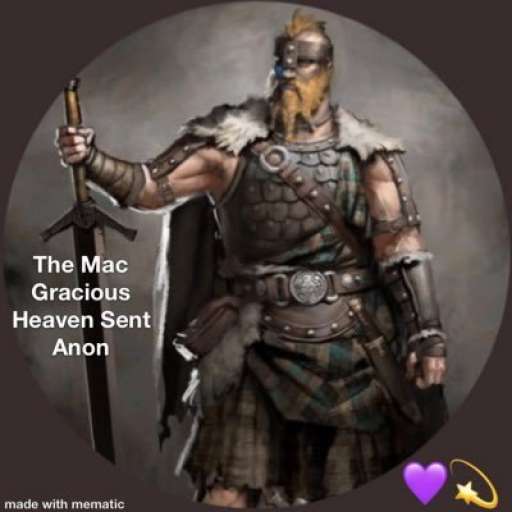
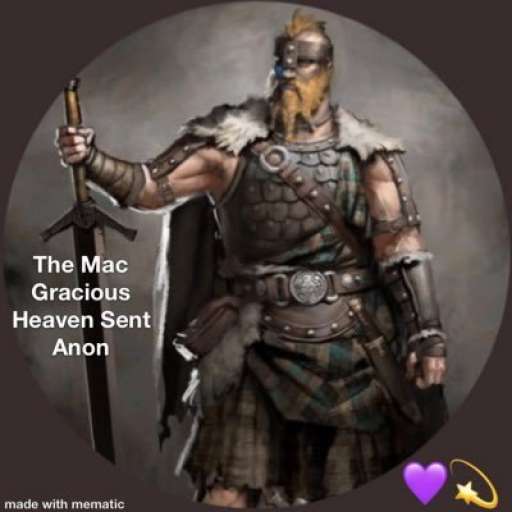
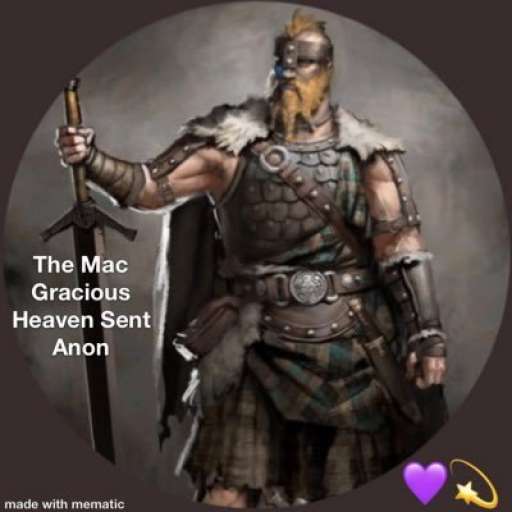
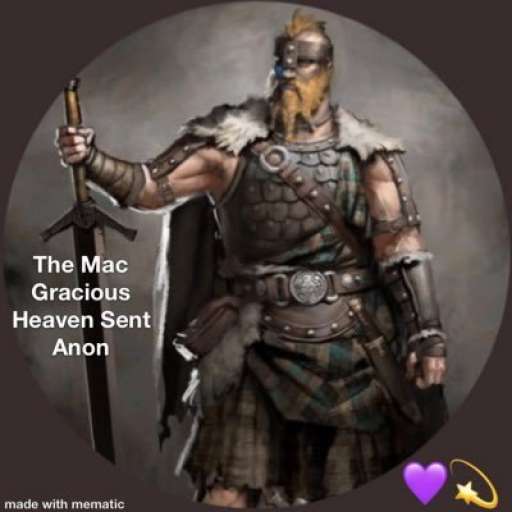
Though DABYCL works well in Molecular Beacons (structured probes with paired reporter and quencher that utilize static quenching), its absorption maximum of 474 nm places it below the maxima of the reporters shown in Figure 2, therefore it is a poor choice for Dual-Labeled Probes (linear probes with separated reporter and quencher that utilize FRET quenching).
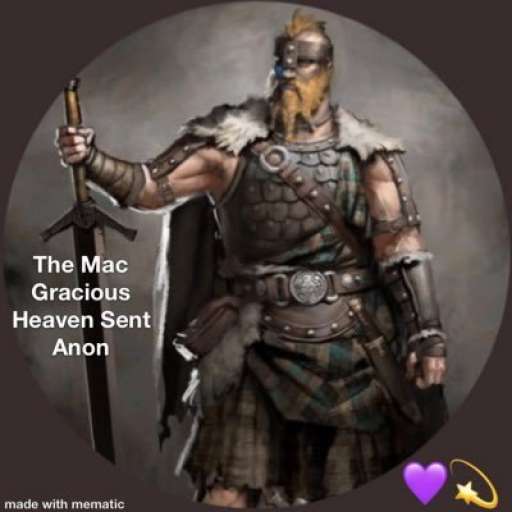
A Molecular beacon is a single-stranded bi-labeled fluorescent probe held in a hairpin-loop conformation (around 20 to 25 nt) by complementary stem sequences (around 4 to 6 nt) at both ends of the probe. The 5’ and 3’ ends of the probe contain a reporter and a quencher molecule, respectively. The loop is a single-stranded DNA sequence complementary to the target sequence. The proximity of the reporter and quencher causes the quenching of the natural fluorescence emission of the reporter. The structure and mechanism of a molecular beacon is shown below.
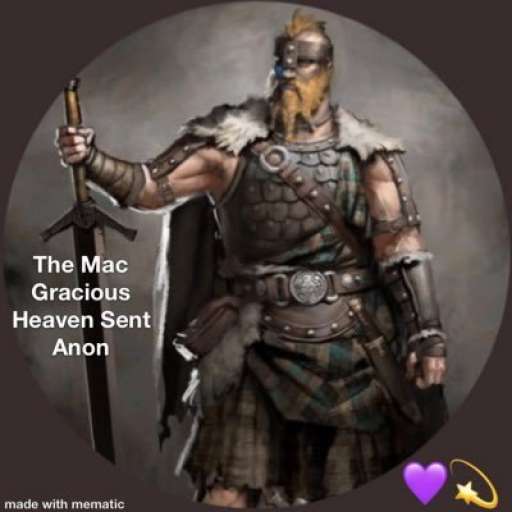
Molecular beacons hybridize to their specific target sequence causing the hairpin-loop structure to open and separate the 5’ end reporter from the 3’ end quencher. As the quencher is no longer in proximity to the reporter, fluorescence emission takes place. The measured fluorescence signal is directly proportional to the amount of target DNA.
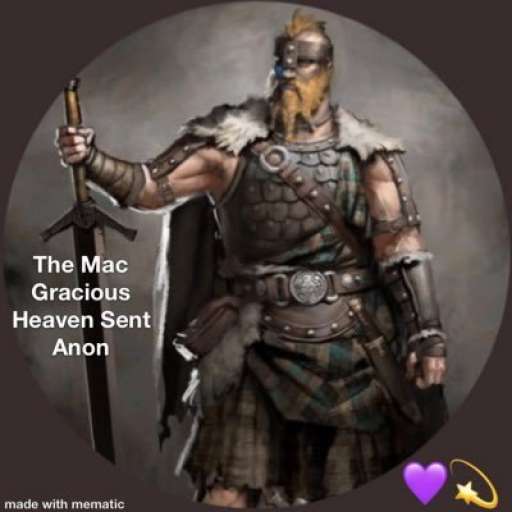
Three molecular beacon FRET pairs were designed, synthesized and tested in solution. These molecular beacons were designed to hybridize to adjacent regions on an mRNA target so that the two fluorophores will lie within the FRET range (∼6 nm) when probe/target binding occurs for both beacons.
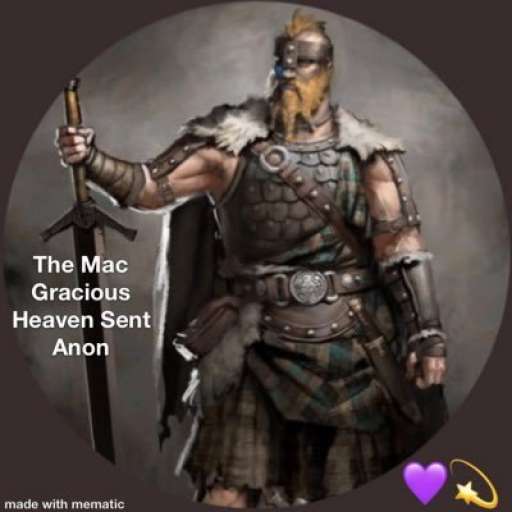
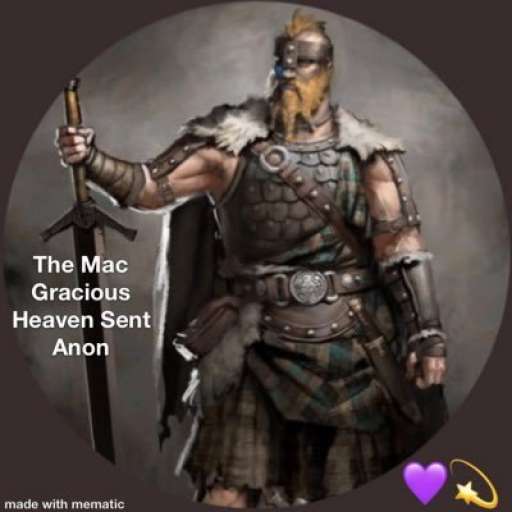
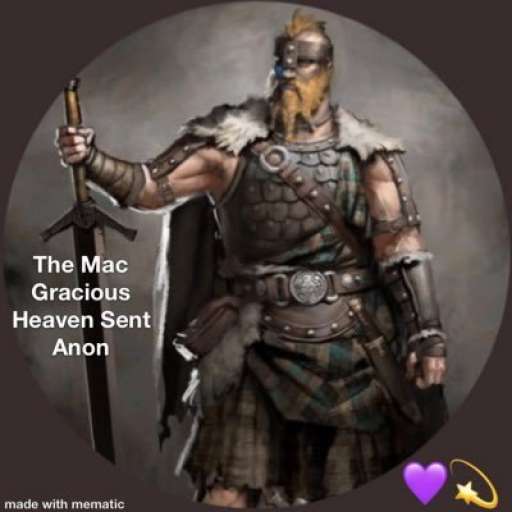
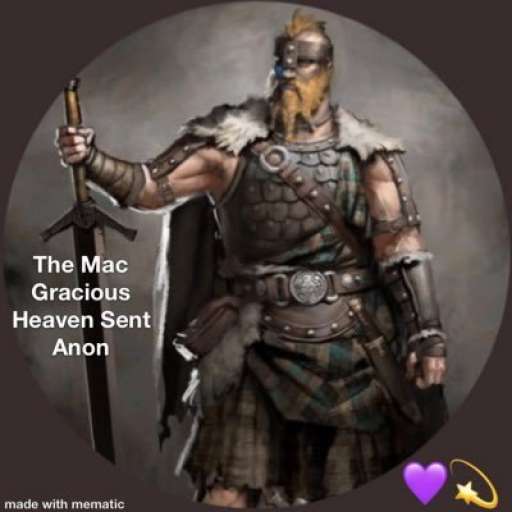
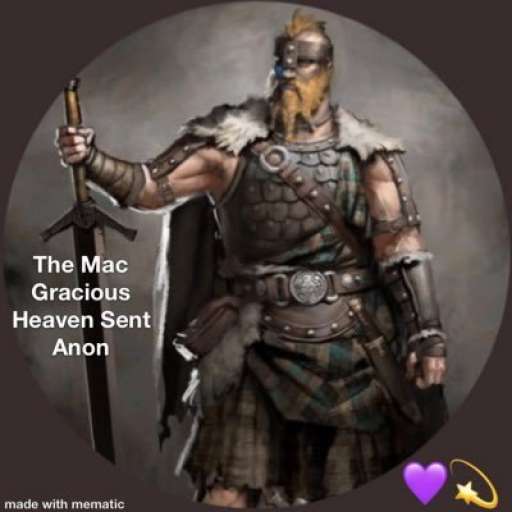
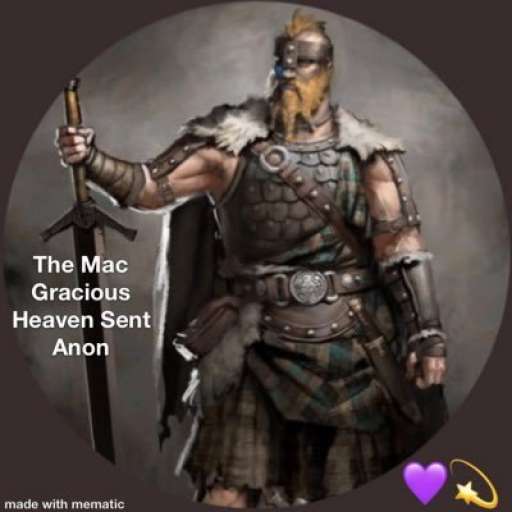
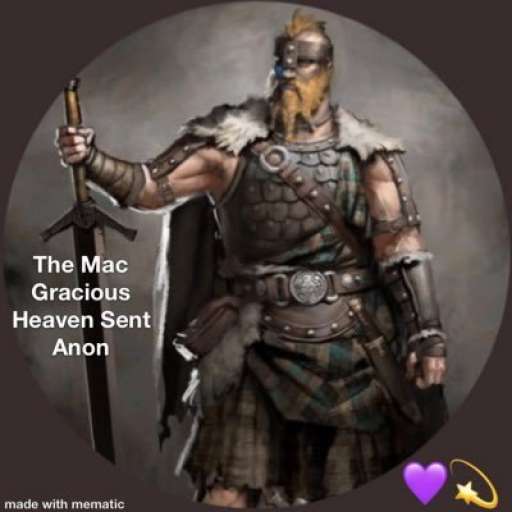
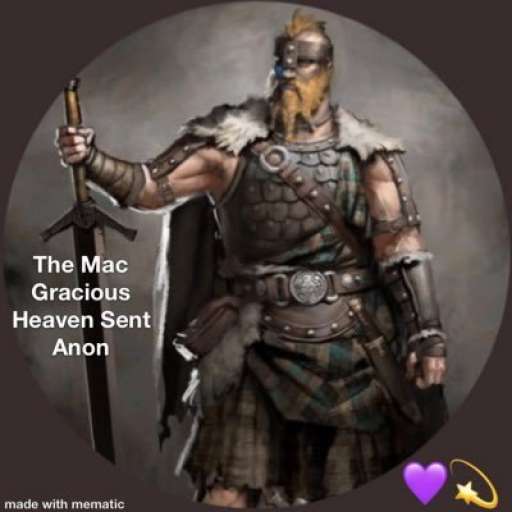
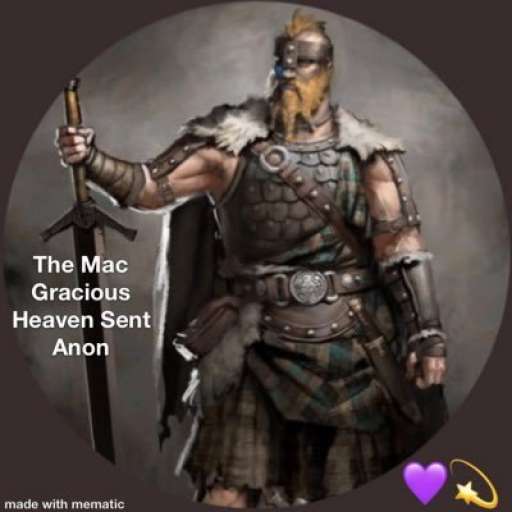
No threat?