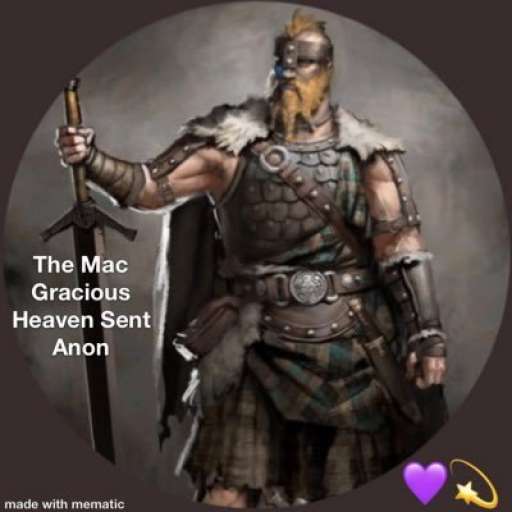
Scientists have discovered how naturally occurring but unstable molecules, known as free radicals, can control the fundamental process of cell division, which, when it goes wrong, can lead to uncontrolled cell growth and cancer.
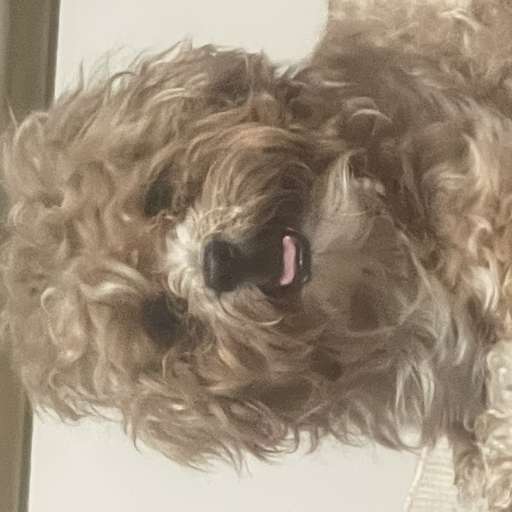
Grail hunter Believer Hierophant Uncompromising scientist There’s something way better than ivermectin
no no no thats not what causes cancer!
There is a specific specific event down to the electron that causes cancer in every type.its the same event my friend..
correlation is not causation.
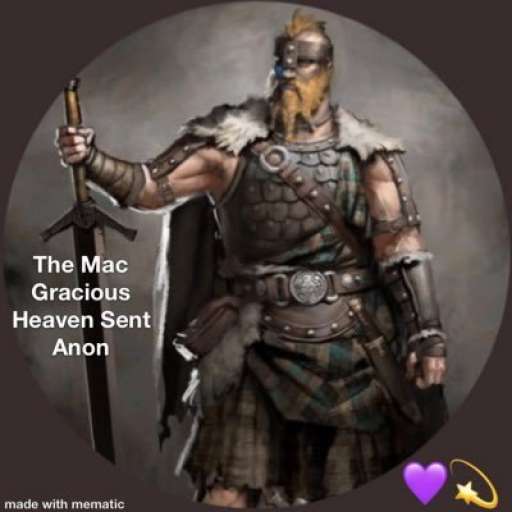
Ionization is the process by which ions are formed by gain or loss of an electron from an atom or molecule. If an atom or molecule gains an electron, it becomes negatively charged (an anion), and if it loses an electron, it becomes positively charged (a cation). Energy may be lost or gained in the formation of an ion.
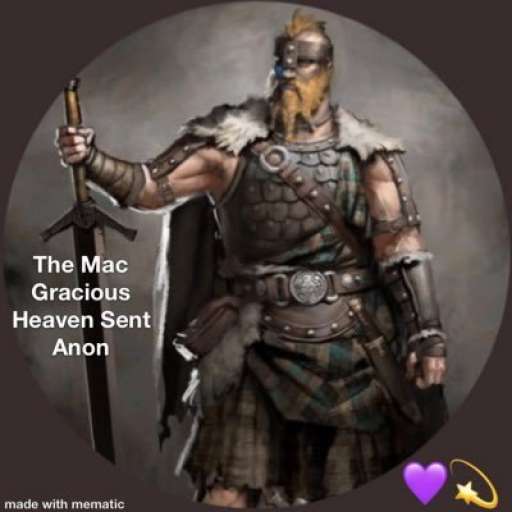
Ions are atoms (or groups of atoms) which carry an electric charge because they have either gained or lost one or more electrons. If an atom gains electrons it acquires a negative charge. If it loses electrons, it becomes positively charged.
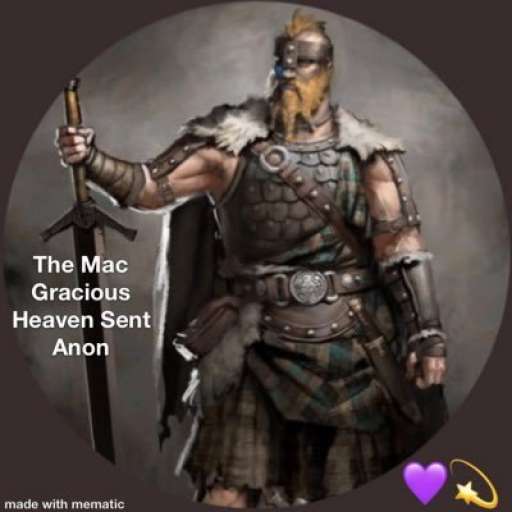
electron, lightest stable subatomic particle known. It carries a negative charge of 1.602176634 × 10−19 coulomb, which is considered the basic unit of electric charge.
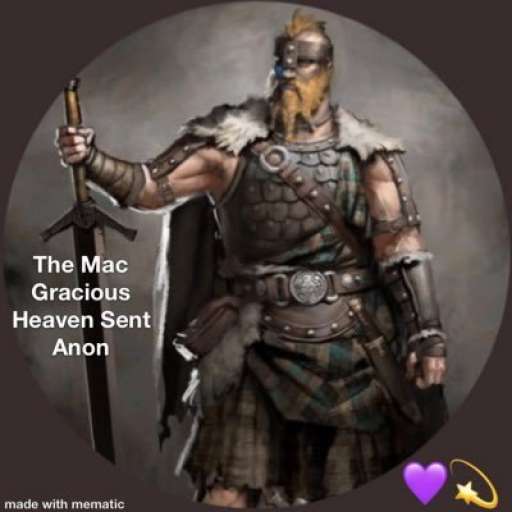
Navy researchers have developed a nanoparticle-based electron donor-acceptor bioconjugate for the real-time perception of changes in cellular membrane potential. It consists of a photoluminescent nanoparticle electron donor, a multidomain membrane insertion peptide, and an electron acceptor. The rate of electron transfer between the donor and acceptor is modulated by changes in membrane potential, which lead to measurable alterations in donor photoluminescence. In one specific case of the donor-acceptor bioconjugate, the electron donor is a semiconductor quantum dot that is connected to fullerene, the electron acceptor, through a membrane-insertion peptide.
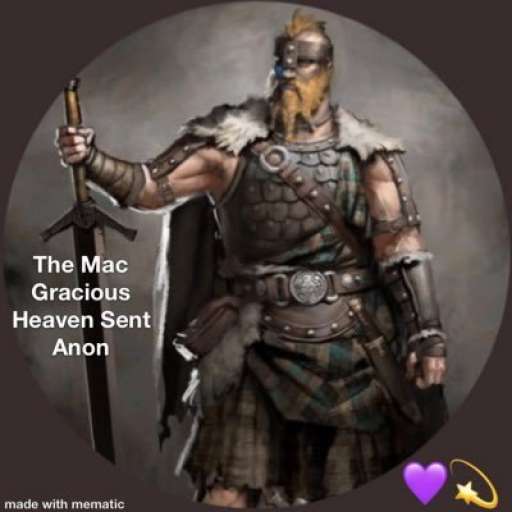
The interactive effects between electron donor substances and iron (Fe) oxides have significant influence on electron transfer and the growth of Fe-reducing bacteria, which may affect the reductive dechlorination of 1,1,1-trichoro-2,2-bis(p-chlorophenyl)ethane (DDT) in soils.
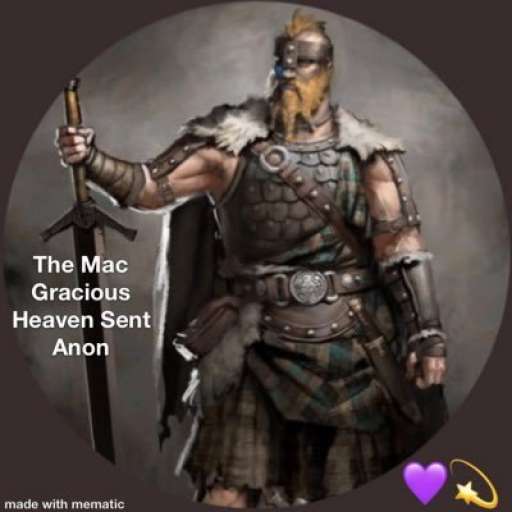
The surfaces of iron oxide nanoparticles are capable of catalytically generating reactive oxygen species (ROS) through the Fenton and Haber-Weiss reactions.
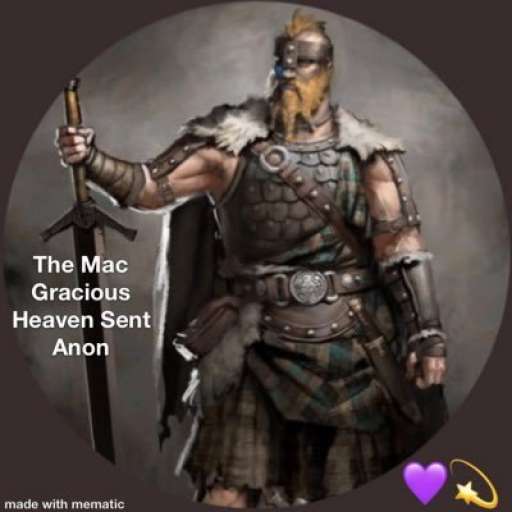
Elevated rates of reactive oxygen species (ROS) have been detected in almost all cancers
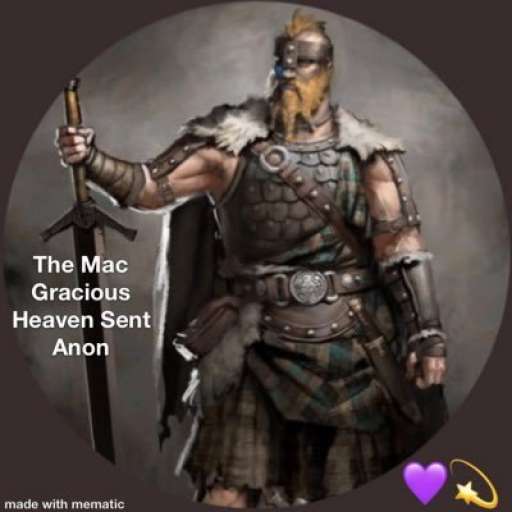
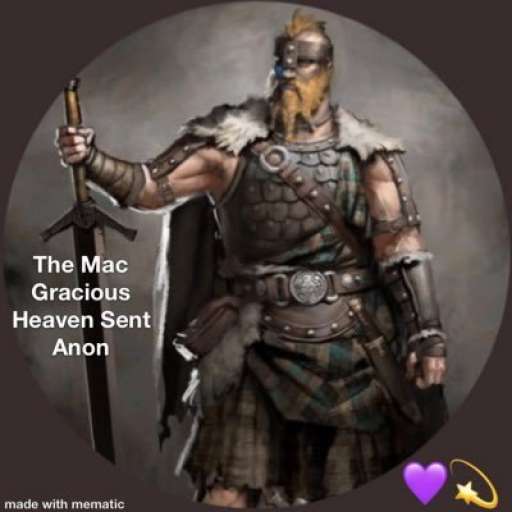
Sonocrystallization involves the application of ultrasound energy to control the nucleation and crystal growth of a crystallization process.
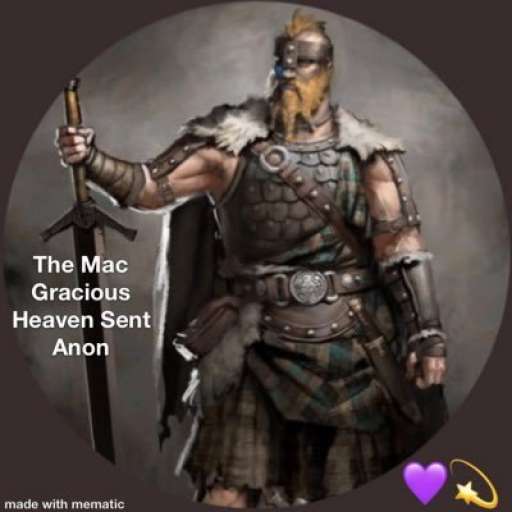
Crystallization refers to the mechanism by which viral components are converted into structured solid particles. They can store these crystallized viruses for many years.
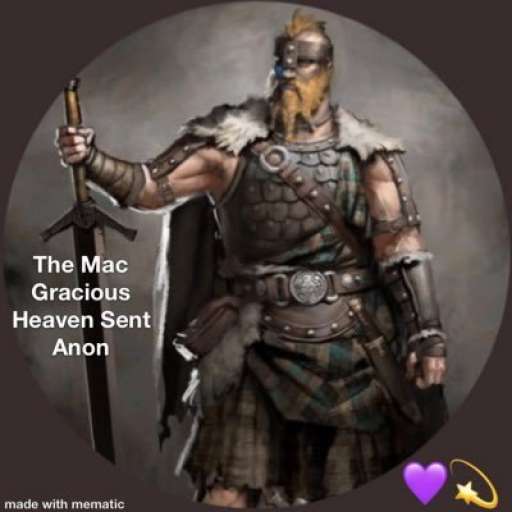
Lipid crystallization is ubiquitous in nature, observed in biological structures as well as in commercial products and applications. In a dehydrated state most of the lipids form well ordered crystals, whereas in an aqueous environment they self-assemble into various crystalline, liquid crystalline or sometimes macroscopically disordered phases. Lipid self-organization extends further to hierarchical levels including structured emulsions and nanostructured particles.
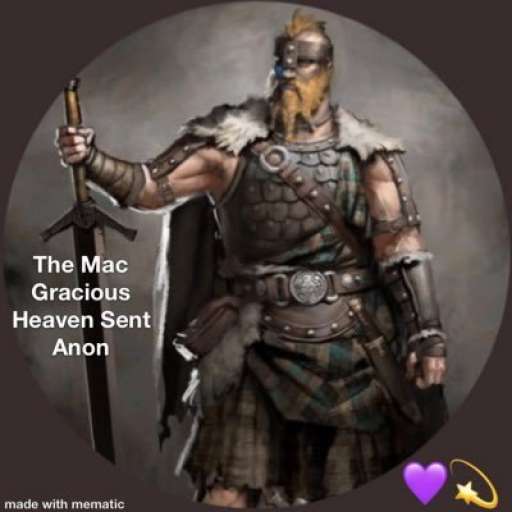
Lipids comprise a diverse group of compounds that include fats, waxes, sterols, phospholipids and many others. The functions associated with lipids are just as diverse, and include membrane structure, signaling, and energy storage. While most lipids are composed of non-polar hydrocarbon structures, other lipids can contain positively and/or negatively charged elements, the nature of which imparts particular physical properties that give charged lipids structural and functional versatility. This page will describe the structure and function of some of the charged lipids commonly encountered in biology.
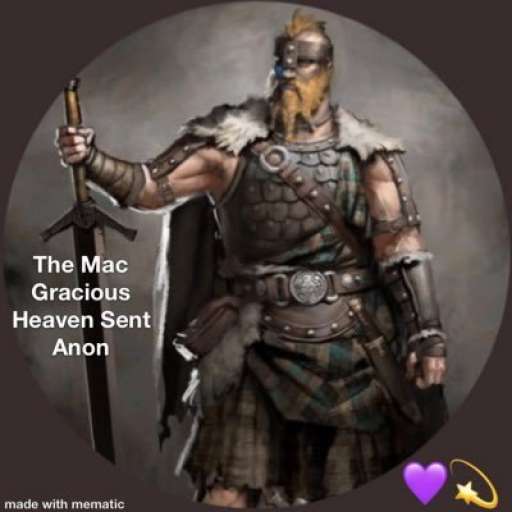
It has been shown that monovalent lipids, including PS and polyvalent phospholipids—PIP2 and PIP3—are asymmetrically distributed on the cytosolic surface of membranes and confer a negative charge to the plasma membrane, creating an electric field equivalent to 105 V/cm [2–5].
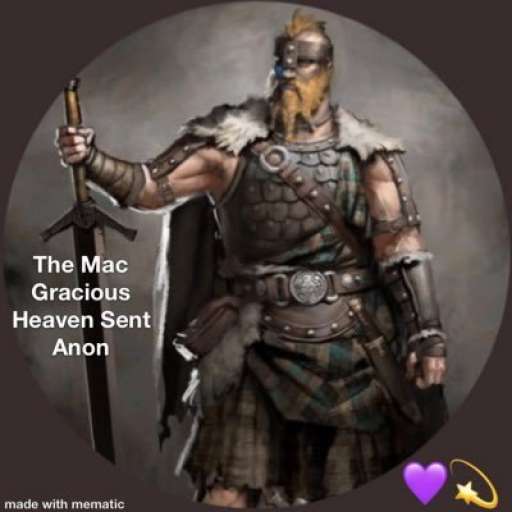
When an electric field is applied to ions in a medium, a phenomenon causes the ions to move with the electric field. The motion of the ion itself can be characterized by charge, shape, and size.
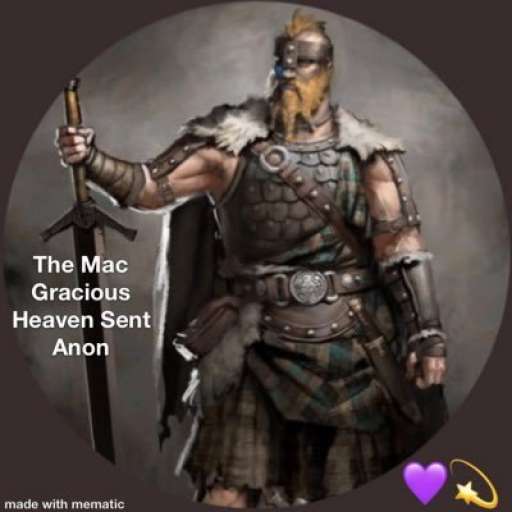
An electron generates an electric field that exerts an attractive force on a particle with a positive charge, such as the proton, and a repulsive force on a particle with a negative charge. The strength of this force in nonrelativistic approximation is determined by Coulomb's inverse square law.
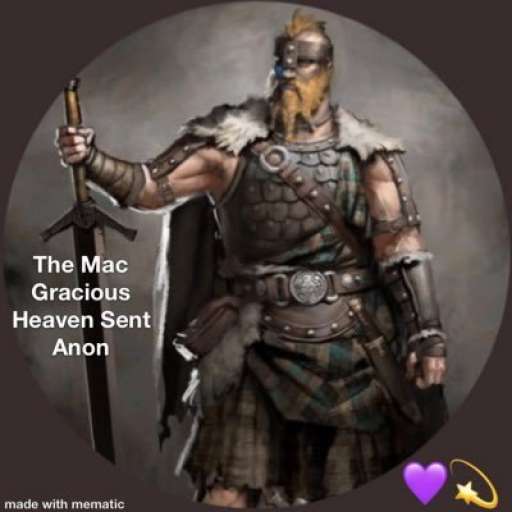
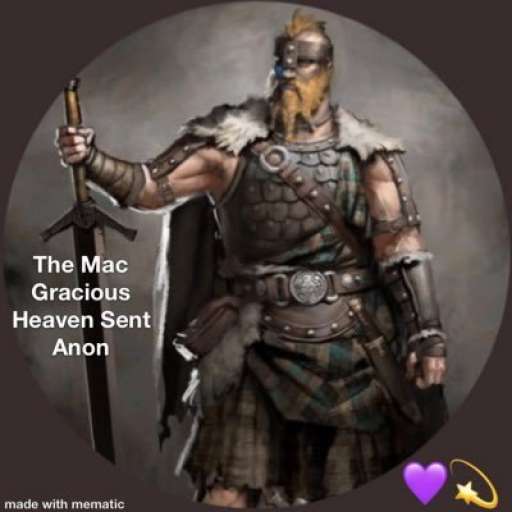
Transport phenomena in a specially optimized vertical asymmetric sub-micron
double barrier structure with modulation-doped barriers is investigated by applying a bias to a special Schottky side gate, which allows the effective area of the conducting channel to be finely “tuned”. For a sufficiently small device, the electrical properties of the controllable quantum dot bounded by well defined heterostructure barriers and an adjustable side wall potential are expected to be governed by single electron charging, and single electron resonant tunneling. Single electron transistor (SET) operation is possible because the number of electrons in the dot, n, can be varied one-by-one with the side gate.
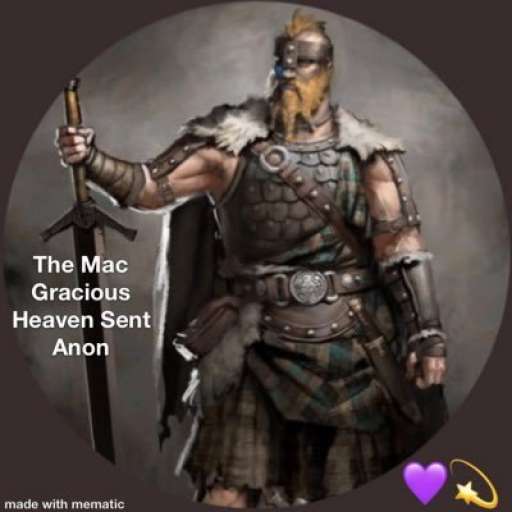
The drain current flowing through the conducting channel in response to a small drain voltage is strongly modulated by the gate voltage close to “pinch-off” as n approaches zero, and oscillations in the drain current persist up to about 25 K. A gate modulated zero current region of Coulomb blockade, step-like features, and resonances exhibiting negative differential resistance are clearly observed at low bias. Although this technology is very promising for the realization of SET operation at temperatures well above 4.2 K, the temperature dependence of the Coulomb blockade is an important limitation.
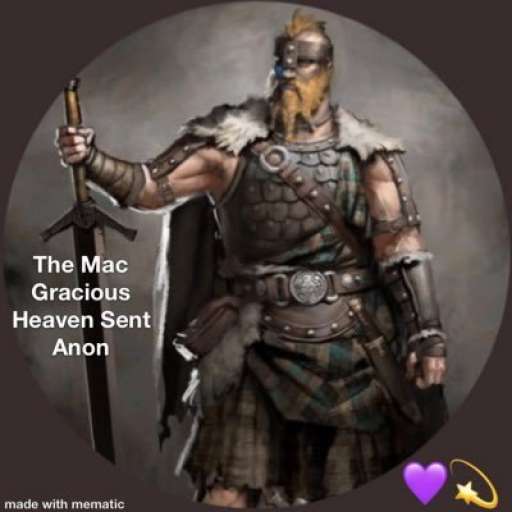
We describe the evolution of the conductance oscillations and the degradation of Coulomb blockade with temperature from 0.3 K up to 25 K, and propose that co-tunneling in a small system containing just a “few” electrons in which the zero-dimensional energy level spacing is significant and comparable to the Coulomb charging energy is important, and is likely to be more complex than that documented for large planar dot structures containing “many” electrons.
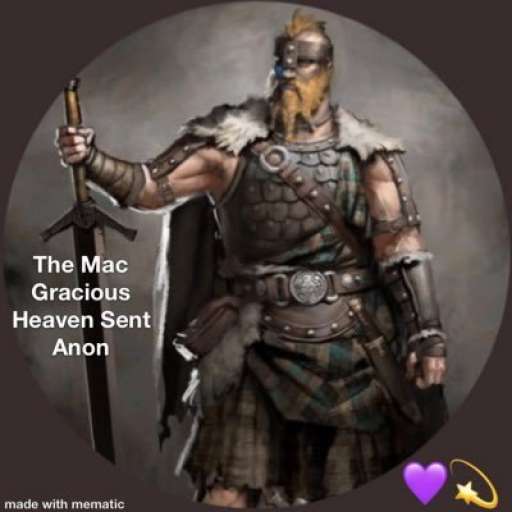
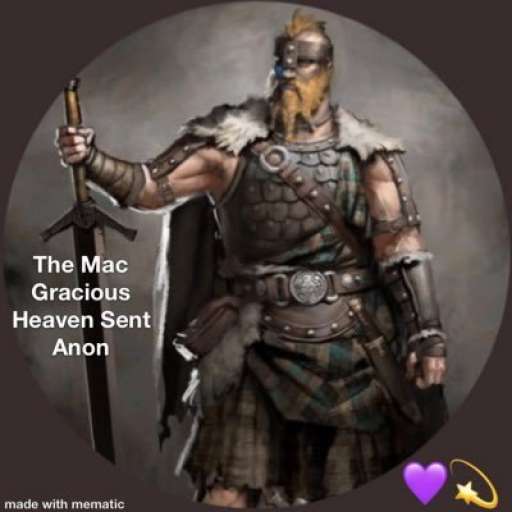
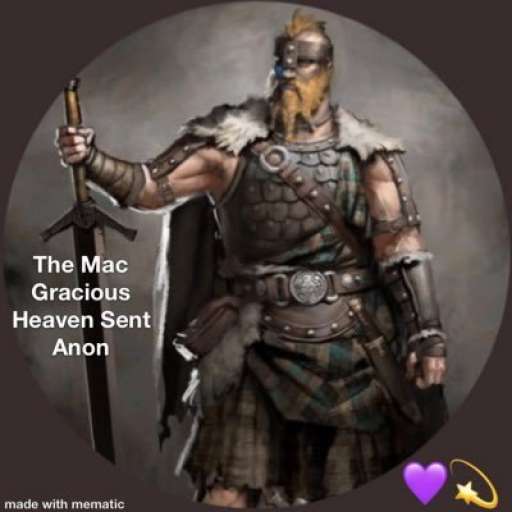
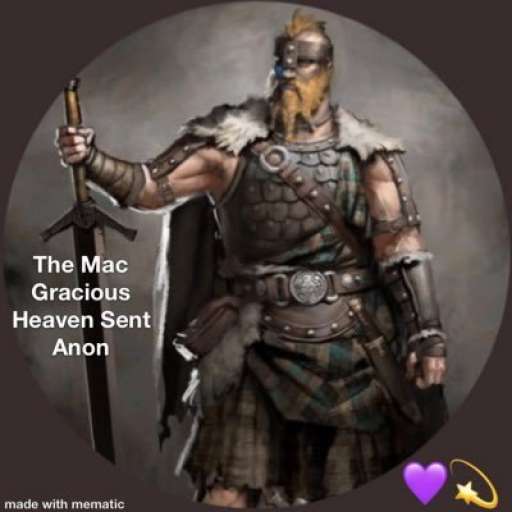
Conceptual idea of strong exciton–phonon proximity coupling a Illustration of interlayer exciton–phonon coupling at the atomic interface of a TMD/gypsum heterostructure. The transient dipole field (magenta curves) of the internal 1s–2p excitonic transition, represented by a snapshot of the exciton wavefunction during excitation (red and blue surface), can effectively couple to the dipole moment of the infrared active vibrational modes in gypsum (red arrows).
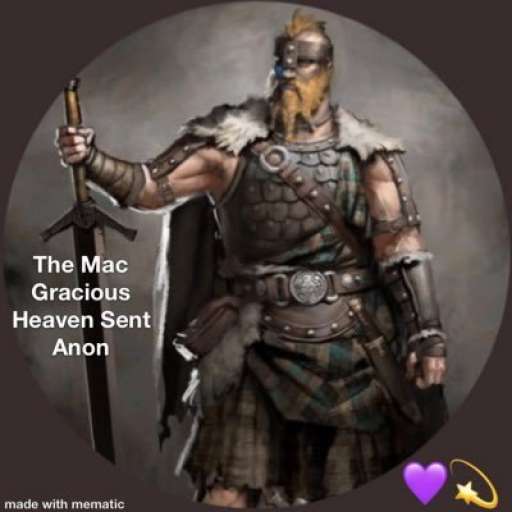
b Optical micrograph of the monolayer WSe2 covered by 100 nm of gypsum. Clear photoluminescence can be observed in WSe2 (light green area) after photoexciting the heterostructure at a photon energy of 2.34 eV. c Photoluminescence spectrum of a gypsum-covered WSe2 ML on a diamond substrate at 250 K, showing a prominent 1s A exciton resonance at 1.67 eV. d Transmission spectrum of the gypsum layer.